SULFUR DIOXIDE IN WORKPLACE ATMOSHPERES (IMPREGNATED
ACTIVATED BEADED CARBON)
Method Number: |
ID-200 |
|
|
Matrix: |
Air |
|
OSHA Permissible Exposure Limits: Final
Rule Limits: |
2 ppm Time Weighted Average (TWA) 5 ppm
Short-Term Exposure Limit (STEL) |
|
Transitional Limit: |
5 ppm TWA |
|
Collection Device: |
An air sample is collected using a calibrated sampling
pump and a glass tube containing impregnated activated beaded carbon
(IABC). A prefilter/cassette assembly can be used to collect
particulate, if necessary. |
|
Recommended Sampling Rate: TWA & STEL: |
0.1 liter per minute (L/min) |
|
Recommended Air Volume: TWA:
STEL: |
12 L (0.1 L/min for 120 min) 1.5 L (0.1 L/min for
15 min) |
|
Analytical Procedure: |
The sampling medium is desorbed in 15 mM sodium
hydroxide which contains 0.3 N ( 1%) hydrogen
peroxide. An aliquot of this solution is analyzed as sulfate by ion
chromatography. |
|
Detection Limit:
Qualitative: Quantitative: |
0.004 ppm (12-L air sample) 0.032 ppm (1.5-L air
sample) 0.013 ppm (12-L air sample) 0.104 ppm (1.5-L air
sample) |
|
Precision and Accuracy: |
TWA
|
STEL
|
Validation Range:
CVT (pooled):
Bias: Overall Error: |
1.36 to 4.16
ppm 0.048 -0.033 ±12.9%
|
5.79 ppm
0.028
(CV2) +0.006
±6.2% |
|
Method Classification: |
Validated Method |
|
Chemist: |
James C. Ku |
Date: |
April, 1992 |
Branch of Inorganic Methods Development OSHA Salt Lake
Technical Center Salt Lake City, Utah
Commercial manufacturers and products mentioned in this method
are for descriptive use only and do not constitute endorsements by
USDOL-OSHA. Similar products from other sources can be
substituted.
1. Introduction
This method describes the sample collection and analysis of airborne
sulfur dioxide (SO2). Samples are taken in the
breathing zone of workplace personnel, and analysis is performed by ion
chromatography (IC).
1.1. History
Previously, OSHA collected compliance samples for
SO2 exposure determinations in
midget-fritted glass bubblers containing 0.3 N ( 1%) hydrogen peroxide
(H2O2) which
converted the SO2 to sulfuric acid
(H2SO4). The
amount of sulfate
(SO42-) in the
peroxide solution was measured by IC and gravimetrically converted to
represent the amount of SO2 collected (5.1.).
Because bubblers are inconvenient to use as personal samplers due to
spillage or breakage, it was desirable to develop a
solid-sorbent sampling method. A method for collecting
SO2 was developed, which sampled
SO2 in the air using a sampling tube
containing impregnated charcoal (5.2.,
5.3.).
The chemical(s) used for charcoal impregnation were not specified and
appear to be proprietary; however, the reaction implies an oxidation
of SO2 using a metal hydroxide base. The
impregnated charcoal oxidized SO2 to
SO42-, which was
then desorbed using a weakly basic solution. An aliquot of the
solution was analyzed by IC (5.2.,
5.3.).
Unfortunately, background levels of
SO42- found in the
impregnated charcoal were considered unacceptable, especially when
applying the current Permissible Exposure Limit (PEL) of 2 ppm.
Previously, the OSHA Time Weighted Average (TWA) PEL was 5 ppm
SO2. Because of this contamination and the
PEL reduction, OSHA reinstituted use of the bubbler method listed in
reference 5.1.
until better methodology could be found.
Using the principle applied for the impregnated charcoal collection
of SO2, a new material, impregnated
activated beaded carbon (IABC) was developed. The IABC has a
significantly lower background level of
SO42- (< 3
µg). This current method was evaluated using the IABC as the
collection media.
1.2. Principle
Sulfur dioxide is collected using IABC sorbent which is contained
in a glass tube. The collected SO2 is
converted to sulfite
(SO32-) by the
sorbent and then slowly oxidized to
SO42-. This
oxidation is augmented at the laboratory by addition of a desorbing
solution containing 0.3 N ( 1%)
H2O2 in 15 mM
sodium hydroxide (NaOH) to each IABC sample. The resultant
SO42- is analyzed
by IC using a conductivity detector; a gravimetric conversion is used
to calculate the amount of SO2 collected.
1.3. Advantages and Disadvantages
1.3.1. This method has adequate sensitivity for determining
compliance with the OSHA Short-Term Exposure Limit
(STEL) of 5 ppm and the TWA-PEL of 2 ppm for workplace
exposures to SO2.
1.3.2. The method is simple, rapid, and easily automated.
1.3.3. The
SO42-
contaminant (background) levels of the IABC sorbent are very low
(< 3 µg), especially when compared to the impregnated
charcoal previously used in OSHA Method No. ID-107.
1.3.4. A disadvantage is the need for a desorption efficiency
(DE) correction which is mass-dependent and may be
lot-dependent (also see Sections 3.7.3.
and 4.1.).
1.4. Method Performance
A synopsis of method performance is presented below. Further
information can be found in Section
4.
1.4.1. This method was validated over the concentration range of
1.36 to 4.16 ppm. An air volume of 12 L and a flow rate of 0.1 L/min
were used.
1.4.2. The qualitative detection limit was 0.0187 µg/mL or
0.187 µg (as
SO42-) when
using a 10-mL solution volume. This corresponds to
0.004 ppm SO2 for a 12-L air
volume.
1.4.3. The quantitative detection limit was 0.0624 µg/mL
or 0.624 µg (as
SO42-) when
using a 10-mL solution volume. This corresponds to
0.013 ppm SO2 for a 12-L air
volume. A 50-µL sample loop and a detector
setting of 1 microsiemens (µS) full-scale output
were used.
1.4.4. The sensitivity of the analytical method, whenusing the
instrumental parameters listed in Section
3.6., was calculated from the slope of a linear working range
curve (0.5 to 10 µg/mL
SO42-). The
sensitivity was 2.2 × 107 area units per 1
µg/mL. A Dionex Series 4500i ion chromatograph with AI450
computer software was used (Dionex, Sunnyvale, CA).
1.4.5. This method compared favorably to OSHA Method no. ID-104
(modified) for SO2 (5.1.)
which served as the reference method.
1.4.6. A desorption efficiency (DE) correction is required at
mass loadings up to 400 µg SO2 (see
Sections 3.7.3.
and 4.1.).
1.4.7. The total pooled coefficient of variation
(CVT) for samples taken at about 0.5, 1,
and 2 times the OSHA PEL (1 to 4 ppm for TWA-type
samples) was 0.048. The method exhibited slight negative bias
(-3.3%) for this concentration range after DE
corrections were applied. Other concentration range and TWA results
are shown below:
|
TWA |
STEL |
LOW |
CV |
0.048 |
0.028 |
0.032 |
Bias |
-3.3% |
+0.6% |
-6.5% |
OE |
±12.9% |
±6.2% |
±12.9% |
For the STEL evaluation, 5.32 ppm was used for test atmospheres.
For sampling at a concentration (LOW) near what may be expected in
indoor air monitoring, approximately 0.3 ppm
SO2 was used. Bias and overall error (OE)
values were calculated from those found analytically versus
theoretical (known) values. The theoretical concentrations were
calculated from flows of a certified cylinder of
SO2 and dilution air.
1.4.8. The collection efficiency at 2 times the PEL was 100%.
Samples were collected from a generated test atmosphere of 4 ppm
SO2 for 120 min.
1.4.9. Breakthrough tests were performed at concentrations of
7.20 and 14.8 ppm SO2. No breakthrough was
found for a sampling time of 240 min and an average sample flow rate
of 0.1 L/min.
1.4.10. Samples can be stored at ambient (20 to 25 °C)
temperature for a period of at least 30 days. Storage stability
results show the mean sample recovery after 30 days was within ±10%
of the theoretical calculations. Samples were stored on a laboratory
bench.
1.5. Interferences
1.5.1. Other particulate sulfate compounds and
H2SO4 will
interfere in the analysis of SO2 if they
are collected in the IABC. Particulate and
H2SO4 mist can
be removed from the air during sampling using a modified sampling
device which contains a Teflon® pre-filter
(see Section
2.1.).
1.5.2. Sulfur trioxide gas (SO3), if
present in a dry atmosphere, can give a positive bias in the
SO2 determination.
1.5.3. Any substance that has the same retention time as
SO42-, when
using the ion chromatographic operating conditions described in this
method, is an interference. If the possibility of an interference
exists, changing the separation conditions (column, eluent flow rate
and strength, etc.) may circumvent the problem.
1.6. Source of Exposure
Sulfur dioxide is generated as a by-product from many
industrial processes. These include the smelting of sulfide ores, the
combustion of coal or fuel oils containing sulfur as an impurity,
paper manufacturing, and petroleum refining (5.4.).
1.7. Physical and Chemical Properties (5.4.,
5.5.)
Sulfur dioxide (CAS No.
7446-09-5) |
Chemical formula |
SO2 |
Formula weight |
64.07 |
Melting point |
-72.7 °C |
Boiling point |
-10.0 °C |
Vapor density |
2.3 (air = 1) |
SO2 is a colorless, nonflammable gas with
a characteristic, strong, and suffocating odor. It is soluble in
water, methanol, ethanol, chloroform, ethyl ether, acetic acid, and
sulfuric acid.
1.8. Toxicology (5.6.)
Information listed within this section is a synopsis of current
knowledge of the physiological effects of
SO2 and is not intended to be used as a
basis for OSHA policy.
Sulfur dioxide is intensely irritating to the eyes and respiratory
tract. Workplace exposure to SO2 can cause
both chronic and acute effects. The chronic effects of exposure
include permanent pulmonary impairment, which is caused by repeated
episodes of bronchoconstriction. It has been reported that workers'
exposure to high concentrations of SO2 (80
to 100 ppm) may cause an increased incidence of nasopharyngitis,
shortness of breath on exertion (dyspnea), and chronic fatigue.
Concentrations of SO2 from 2 to 36 ppm
produced a significantly higher frequency of respiratory disease
symptoms, including chronic coughing, expectoration, and dyspnea.
The acute effects include upper respiratory tract irritation,
rhinorrhea, choking, and coughing. Within 5 to 15 minutes from the
onset of exposure, workers develop temporary reflex
bronchoconstriction and increased airway resistance.
2. Sampling
2.1. Equipment
2.1.1. Calibrated personal sampling pumps capable of sampling
within ±5% of the recommended flow rate of 0.1 L/min are used.
2.1.2. Solid sorbent sampling tubes are
prepared using glass tubes, glass wool plugs, and IABC.
Sampling tubes can be commercially obtained. Two types of
sampling tubes are commercially available:
Type I is a glass tube packed with a
100-mg IABC front and 50-mg backup section
(Cat. No. 226-80, SKC Inc., Eighty Four, PA). The IABC
is held in place with glass wool, foam, and a stainless steel
retainer clip. If interference from particulate is probable, a
prefilter/cassette sampling assembly can be used with this tube. See
Section 2.1.5.
for more details regarding the prefilter.
Type II, a combination sampling device (Forest
Biomedical, Salt Lake City, UT) can be used to remove particulate
and collect
H2SO4 mist
during SO2 sampling. The combination
device, as shown below, consists of two different glass tubes
connected together. The front part of the tube contains a
Teflon® filter, retaining rings, foam, and a glass wool
plug. The Teflon® filter is used to trap any particulate
and H2SO4. The
dimensions of the front portion of the sampling device are
12-mm o.d., 10-mm i.d., and
25-mm long. The second part of the device contains two
sections of IABC and is used for collecting
SO2. The dimensions of the second part are
6-mm o.d., 4-mm i.d., and
50-mm long. Both ends of the sampling tube are sealed
with plastic caps.
<---<-----<
Sample Flow <---<-----
Type II -
Combination Sampling Device
If commercial tubes are unavailable, sampling tubes can be
prepared using carbon bead impregnated in the same fashion as
discussed in OSHA method ID-180
for phosphine (5.7.).
Note: The grade of carbon bead appears less significant
for SO2 when compared to phosphine (5.7.).
Prepare each tube for SO2 collection
with a 100-mg IABC front and a 50-mg
backup section. Separate each IABC section using a small amount of
glass wool.
2.1.3. A stopwatch and bubble tube or meter are used to calibrate
pumps.
2.1.4. Various lengths of polyvinyl chloride tubing are used to
connect sampling tubes to pumps.
2.1.5. If the workplace air being sampled is
suspected of containing particulate which could interfere (i.e.
H2SO4 or
sulfates), the prefilter/cassette assembly listed below or a Type II
sampling tube should be used.
- Filter for particulate collection, Teflon® (PTFE),
0.45 µm pore size, 25-mm diameter (part no. 130620,
Nucleopore Corp., Pleasanton, CA)
- Carbon-filled polypropylene cassette, 25-mm
diameter, (part no. 300075, Nucleopore) (See Section 4.10.
for further details regarding this cassette)
- Porous plastic support pad (part no. 220600, Nucleopore)
Note: Do not use glass fiber prefilters for particulate
collection during sampling for SO2. Loss
of SO2 can occur due to the slightly basic
properties of these filters. See Section
4.10. for further details.
Assemble the prefilter assembly such that sampled air enters the
Teflon® filter first and the plastic support pad faces
the sampling tube. Use a minimum amount of tubing to connect the
Type I sampling tube to the prefilter assembly.
2.2. Sampling Procedure (Bulk or wipe samples are not applicable)
2.2.1. Connect the sampling tube (Type I or II) to the
calibrated sampling pump, making sure sampled air enters the large
section (100 mg) of IABC first. Place the sampling device on the
employee such that air is sampled from the breathing zone.
2.2.2. For STEL samples, use a flow rate of 0.1 L/min and a
minimum sampling time of 15 min. For TWA determinations, take
consecutive 12-L samples at a flow rate of 0.1 L/min
for 120 min each. If possible, take enough consecutive samples to
cover the entire work shift.
2.2.3. After sampling, place plastic end caps tightly on both
ends of the tube and apply OSHA Form 21 seals. Record the sampling
conditions.
2.2.4. Use the same lot of IABC tubes for blank and collected
samples. Handle the blank sorbent tube in exactly the same manner as
the sample tubes except that no air is drawn through it. Submit at
least one blank tube for each batch of ten samples.
2.2.5. When other compounds are known or suspected to be present
in the air, such information should be transmitted with the sample.
2.2.6. Specify SO2 analysis and ship
samples to the laboratory. If necessary, any Teflon®
pre-filters used can be analyzed for
H2SO4 (when
H2SO4 is
suspected to be present in the workplace atmosphere).
3. Analysis
3.1. Safety Precautions
3.1.1. Refer to appropriate IC instrument manuals and the
Standard Operating Procedure (SOP) for proper instrument operation
(5.8.).
3.1.2. Observe laboratory safety regulations and practices.
3.1.3. Sulfuric acid, sodium hydroxide, and hydrogen peroxide are
corrosive. Use appropriate personal protective equipment such as
safety glasses, gloves, and lab coat when handling corrosive
chemicals. Prepare solutions in an exhaust hood.
3.2. Equipment
3.2.1. Ion chromatograph (Model 4000i or 4500i Dionex,
Sunnyvale, CA) equipped with a conductivity detector.
3.2.2. Automatic sampler (Dionex Model AS-1) and
sample vials (0.5 mL).
3.2.3. Laboratory automation system: Ion chromatograph interfaced
with a data reduction system (AI450, Dionex).
3.2.4. Micromembrane suppressor, anion (Model AMMS-1, Dionex).
3.2.5. Separator and guard columns, anion (Model HPIC-AS4A and
AG4A, Dionex).
3.2.6. Disposable syringes (1 mL).
3.2.7. Syringe pre-filters, 0.5-µm pore size
(part no. SLSR 025 NS, Millipore Corp., Bedford, MA).
Note: Some syringe pre-filters are not
cation- or anion-free. Tests should be performed with
blank solutions first to determine contamination and suitability
with the analyte.
3.2.8. Miscellaneous volumetric glassware: Micropipettes,
volumetric flasks, Erlenmeyer flasks, graduated cylinders, and
beakers.
3.2.9. Scintillation vials, glass, 20-mL.
3.2.10. Equipment for eluent degassing (vacuum pump, ultrasonic
bath).
3.2.11. Analytical balance (0.01 mg).
3.3. Reagents - All chemicals should be at least reagent grade.
3.3.1. Principal reagents:
CAUTION: NaOH,
H2SO4, or 30%
H2O2 can cause
skin irritation or burns.
Sodium carbonate
(Na2CO3) Sodium
bicarbonate (NaHCO3) Sodium hydroxide
(NaOH) Sulfuric acid
(H2SO4),
concentrated, 98% Hydrogen peroxide
(H2O2),
30% Sodium sulfate
(Na2SO4),
anhydrous Deionized water (DI H2O) with
a conductance of <10 µS.
3.3.2. Eluent (1.0 mM
Na2CO3 + 1.0 mM
NaHCO3):
Dissolve 0.212 g
Na2CO3 and 0.168
g NaHCO3 in 2.0 L DI
H2O, Sonicate this solution and degas
under vacuum for 15 min.
3.3.3. Suppressor regenerant solution (0.02 N
H2SO4):
Carefully transfer 1.14 mL concentrated
H2SO4 into a
2-L volumetric flask which contains approximately 500
mL DI H2O. Dilute to volume with DI
H2O.
3.3.4. Desorbing solution [0.3 N ( 1%)
H2O2 in 15 mM
NaOH]:
Dissolve 0.6 g NaOH in
approximately 500 mL of DI H2O contained
in a 1-L volumetric flask. Carefully add 34 mL of 30%
H2O2 and then
dilute to the 1-L mark with DI
H2O. Prepare weekly.
3.3.5. Sulfate
(SO42-) stock
standard (1,000 µg/mL):
Dissolve and dilute 1.4792 g of
Na2SO4 to 1.0 L
with DI H2O. Prepare yearly.
3.3.6. Sulfate
(SO42-) standard
solutions, 100, 10, and 1 µg/mL:
Pipette appropriate volumes of the 1,000 µg/mL
SO42- stock
standard into volumetric flasks and dilute to the mark with eluent.
Prepare monthly.
3.4. Working Standard Preparation
3.4.1. Prepare
SO42- working
standards in eluent. A method for preparing a series of working
standards using 10-mL final solution
volumes is shown below:
Working Std (µg/mL)
|
Std Solution (µg/mL)
|
Aliquot (mL)
|
Eluent Added (mL)
|
0.5 1 2 5 10 20 30
50 |
1 1 10 10 10 100
100 100 |
5 * 2 5 * 2 3 5 |
5 * 8 5 * 8 7 5 |
* Already prepared
in Section 3.3.6. |
3.4.2. To prepare each working standard listed above, pipette an
appropriate aliquot of the specified standard solution (prepared in
Section
3.3.6.) and add the specified amount of eluent.
3.4.3. As an alternative, pipette each aliquot into a
10-mL volumetric flask and dilute to volume with
eluent.
3.5. Sample Preparation
Note: If
H2SO4 is a
requested analyte and a Type II sampling device or a PTFE prefilter
was used, see OSHA Stopgap Method ID-165SG
or OSHA Method No. ID-113 for further details regarding
sample preparation, analysis, and calculation of results for
H2SO4.
3.5.1. Carefully remove and discard the rear glass wool plug (or
foam for the Type II sampler) without losing any beaded
carbon.
Note: The sorbent should always be removed from the glass
tube via the opposite end of collection (i.e. 50-mg
IABC backup section is removed first). This will minimize the
possibility of contamination from any collected particulate.
3.5.2. Carefully transfer each IABC section from a sample tube
and place in separate 25-mL Erlenmeyer flasks or
scintillation vials.
3.5.3. Pipette 10 mL of desorbing solution
into each flask. Cap each flask tightly and allow each solution to
sit for at least 60 min. Occasionally swirl each solution.
3.6. Analysis
3.6.1. Pipette a 0.5- to 0.6-mL portion of each standard or
sample solution into separate automatic sampler vials. Place a
filtercap into each vial. The large filter portion of the cap should
face the solution.
3.6.2. Load the automatic sampler with labeled samples,
standards, and blanks.
3.6.3. Set up the ion chromatograph in accordance with the SOP
(5.8.)
Note: An SOP is a written procedure for a specific
instrument. It is suggested that SOPs be prepared for each type of
instrument used in a lab to enhance safe and effective operation.
Typical operating conditions for a Dionex 4000i or 4500i with a
conductivity detector and an automated sampler are listed below:
Ion Chromatograph
|
Eluent: |
1.0 mM
Na2CO3/1.0
mM NaHCO3 |
Column temperature: |
ambient |
Anion precolumn: |
AG4A |
Anion separator column: |
AS4A |
Anion suppressor: |
AMMS-1 |
Conductivity Output range: |
1 µS |
Sample injection loop:
|
50 µL
|
Pump
|
Pump pressure: |
900
psi |
Flow rate:
|
2 mL/min
|
Chromatogram
|
Run time: |
10 min |
Peak retention time: |
6 min for
SO42- |
3.6.4. Follow the SOP for further instructions regarding analysis
(5.8.).
3.7. Calculations
3.7.1. After the analysis is completed, retrieve the peak areas
or heights. Obtain hard copies of chromatograms from a printer.
3.7.2. Prepare a concentration-response curve by
plotting the peak areas or peak heights versus the concentration of
the SO42-
standards in µg/mL.
3.7.3. Perform a blank correction for each
IABC front and backup sections. Subtract the µg/mL
SO42- blank
value (if any) from each sample reading if blank and sample solution
volumes are the same. If a different solution volume is used,
subtract the total µg blank value from total µg sample
values.
3.7.4. Calculate the air concentration of
SO2 (in ppm) for each air sample:
A = (µg/mL
SO42-) × (Sol
Vol) × (GF)
ppm SO2 = |
(corr µg
SO2) × (Mol Vol)
(AV) × (Mol Wt) |
Where:
|
A |
= |
uncorrected µg SO2 |
corr µg SO2 |
= |
µg SO2 with DE correction
applied |
µg/mL
SO42- |
= |
Amount found (from calibration curve) |
Sol Vol |
= |
Solution volume (mL) from Section
3.5.3. |
GF,
SO2/SO42- |
= |
Gravimetric factor = 0.667 |
Mol Vol |
= |
Molar volume (L/mol) = 24.45 (25 °C and 760
mmHg) |
AV |
= |
Air volume (L) |
Mol Wt |
= |
Molecular weight for SO2
= 64.0 (g/mol) |
DE |
= |
Desorption efficiency (mass-dependent, see
sliding scale below or equation in 3.7.4.) |
Amt of SO2 (µg)
|
DE Factor
|
<
30 31 51 76 101 201 >400 |
to to to to to |
50 75 100 200 400 |
|
0.800 0.825 0.850 0.875 0.900 0.950 1.000 |
3.7.5. An alternative to the DE correction sliding
scale above is the following equation:
DE = -1.1386 ×
10-6 (A)2
+ 1.0037 × 10-3
(A) + 7.81 × 10-1
Where: A = uncorrected µg
SO2
3.7.6. The DE correction may be lot-dependent. The
corrections stated in this method were determined using lot no. 673
(Cat. no. 226-80, SKC Inc., Eighty Four, PA) or beaded
carbon prepared for sampling phosphine (5.7.).
A difference in DE was not noted for these two preparations (Section
4.1.2.). Future lots or different grades should be evaluated for
DE corrections.
3.8. Reporting Results
Add the backup section ppm SO2 result (if
any) to the front section result for each sample. Report results to
the industrial hygienist as ppm SO2.
4. Backup Data
This method has been validated for a 12-L,
120-min sample taken at a flow rate of 0.1 L/min. The method
validation was conducted near the OSHA TWA-PEL and STEL of 2
ppm and 5 ppm, respectively. The sampling tubes used during the validation
consisted of a two-section tube (Type I) packed with a
100-mg IABC front and 50-mg backup section or
Type II (see Section
2.1.2). Tubes were obtained commercially (Lot no. 673, Cat. no.
226-80, SKC Inc., Eighty Four, PA) or prepared
in-house. Preliminary tests were conducted using a 10% (w/w)
impregnation of a metal hydroxide base on the carbon bead; however, tests
conducted at high humidity (80%) indicated a formation of a slurry inside
the sampling tube. The amount of metal hydroxide base was lowered to 1%
during validation. A difference in SO2 results
using either 1 or 10% base impregnation was not noted; an exception was
the 80% RH tests where slurry formation made it difficult to remove the
carbon bead from the glass tubes.
The validation consisted of the following experiments:
- An analysis of 19 samples (6 samples each at 2 × and 1
× TWA-PEL, and 7 samples at 0.5 ×
TWA-PEL) for the DE study.
- A sampling and analysis of 16 samples (6 samples each at 2
× and 1 × TWA-PEL, and 4 samples at
0.5 × TWA-PEL) collected from dynamically
generated test atmospheres at 50% RH. Samples at a concentration near
the STEL and at concentrations expected during indoor air quality
investigations (
0.3 ppm) were also
taken.
- A determination of the sampling media collection efficiency at
approximately 4 ppm (2 × TWA-PEL).
- A determination of breakthrough.
- An evaluation of storage stability at 20 to 25 °C for 24 collected
samples.
- A determination of any significant effects on results when sampling
at different humidities.
- A determination of the qualitative and quantitative detection
limits.
- A comparison of methods.
- Evaluation of the Type II sampling tube for collecting
SO2.
- Evaluation of a prefilter/cassette assembly for use with Type I
samplers.
- Summary.
All theoretical (known) concentrations of generated test atmospheres
were calculated from controlled flows of a cylinder of 303 ppm
SO2 in nitrogen (certified concn, Alphagaz,
LaPorte, TX) and dilution air. An analysis of the cylinder concentration
using OSHA Method No. ID-104
(modified) (5.1.)
indicated the manufacturer's stated concentration was accurate. The OSHA
method ID-104 was modified such that the 0.3 N
H2O2 sample collection
solution was made more basic by addition of NaOH. The final collection
solution used for the modified method ID-104 was 0.3 N
H2O2 in 15 mM NaOH.
A generation system was assembled, as shown in Figure
1, and used for all experiments except detection limit determinations.
Samples using OSHA Method ID-104 (modified) were taken
side-by-side with any IABC samples. All samples were analyzed
by IC. The IABC samples were subject to the sliding scale DE
corrections listed in Section
3.7.3 (with the obvious exception of those samples used to
calculate DE corrections in Section
4.1.). A correction was not applied nor necessary for the liquid
sorbent sampler results (ID-104 modified), or sulfate
solutions used to calculate detection limits.
All results were calculated from concentration-response curves and
statistically examined for outliers. In addition, the analysis (Section
4.1.) and sampling and analysis results (Section
4.2.) were tested for homogeneity of variance. Possible outliers were
determined using the Treatment of Outliers test (5.9.).
Homogeneity of variance was determined using the Bartlett's test (5.10.).
Statistical evaluation was conducted according to Inorganic Methods
Evaluation Protocol (5.11.).
The overall error (OE) (5.11.)
was calculated using the equation:
OEi = ± (
|biasi| +
2CVi) × 100% (95% confidence level)
Where i is the respective sample pool being examined.
4.1. Analysis
Nineteen samples were prepared by adding known amounts of
SO2 to the IABC tubes to determine
recoveries (DE) for the analytical portion of the method. For this
experiment, an active method of spiking with low-flow
(0.03 L/min) sampling pumps was used to determine the amount of gas
collected and not necessarily the sampling capability of the IABC at
the low-flow rate.
4.1.1. Procedure: Sampling tubes containing IABC
were spiked using the low flow pumps and generation system as
described in references 5.7.
and 5.12.
The SO2 source mentioned previously was
diluted to approximately 30 ppm using the generation system shown in
Figure
1. Calibrated low-flow-rate pumps were connected to
the sampling manifold and were used to deliver the spikes for
measured time periods. Air used to dilute the
SO2 source was tempered to 50% RH and 25
°C. Pumps used for this experiment (Miniature Personal Air Sampling
Pumps, Model No. 222-3-12, SKC Inc., Eighty Four, PA)
were calibrated to collect samples at 0.030 L/min. Spikes were
approximately 32, 64, 130, and 400 µg
SO2. These levels correspond approximately
to 0.5, 1, 2, and 6 times the PEL for a 12-L air sample
at a 0.1-L/min flow rate.
4.1.2. Results: Desorption
efficiencies, presented in Table
1, varied depending on the amount of
SO2 collected. For 0.5 times the PEL (32
µg SO2), the DE was close to 0.8;
the DE was 1.0 for 6 × PEL ( 400 µg SO2). The DE
corrections are similar (although larger) to those found during a
previous validation of a sorbent tube (Method no. ID-107, see
reference 5.3.)
for SO2 collection. The previous method
used charcoal impregnated in a fashion similar to the IABC sorbent.
The average DE correction for the lot of treated charcoal specified
in Method no. ID-107 was 0.927, and resulted from
averaging DEs of 0.909 (168 µg
SO2), 0.934 (333 µg
SO2), and 0.940 (669 µg
SO2).
A mechanism to explain the decrease in DE at low mass loadings
has not been found; however, it is possible that a portion of the
initial SO2 entering the sampling tube
strongly bonds with the surface of the carbon bead matrix and
becomes unrecoverable. A strong and weak bonding mechanism for
SO2 has been noted on active carbon
surfaces (5.13.).
Once the strong bonding sites are occupied, a DE closer to what is
expected is achieved. At larger mass loadings the amount of strongly
bonded SO2 would remain the same, and
become insignificant when compared to the total amount collected;
thus the DE approaches unity.
A test of two different grades of beaded carbon (impregnated) was
also conducted and a significant difference in DE was not noted:
(Sampling Time = 120 min, flow rate 0.1 L/min, SO2
concentration = 1.19 ppm, 25 °C, 50% RH)
|
SKC Bulk
|
SKC lot no. 745*
|
Grade Sample Type N Mean (ppm
SO2) Std Dev (ppm
SO2) CV Recovery |
General II 3 1.19** 0.015 0.013 100% |
MU-AZ II 3 1.20** 0.006 0.005 101% |
* |
SKC Catalog no. 226-32 (These tubes were
manufactured for the collection of phosphine. The
appropriate amount of IABC was removed from a tube, placed
in a Type II sampling device, and used to collect
SO2). |
** |
DE corrected using scale shown in Section
3.7.3. |
Grades listed above are assigned by the manufacturer of the
untreated beaded carbon (Kureha Chemical, NY) to designate bead type
and manufacturing process used. The general grade is a more common
bead which was found to have poor retention efficiency for
collection of phosphine (5.7.).
Although the data shows comparable DEs, even for different
grades, future lots or grades of beaded carbon should be evaluated
for DE corrections.
4.2. Sampling and Analysis
To determine the precision and accuracy of the method, known
concentrations of SO2 were generated,
samples were collected, prepared, and analyzed.
4.2.1. Procedure:
- The SO2 source mentioned previously
was used to generate test atmospheres of
SO2. This source was diluted with
filtered, humidified air using the system shown in Figure
1.
- Dynamic generation system
A Miller-Nelson
Research Inc. flow, temperature, and humidity control system
(Model HCS-301, Monterey, CA) was used for air flow
control and conditioning. All generation system fittings and
connections were Teflon®. A glass mixing chamber was
used to mix the tempered, filtered air with the contaminant gas.
The system was set to generate test atmospheres at 50% RH and 25
°C.
- The SO2 and diluent air flow rates
were adjusted using mass flow controllers. Flow rates were also
measured using a dry test meter (for diluent air) and a soap
bubble flow meter (for SO2 gas).
- Samples were taken from the sampling manifold using
constant-flow pumps. Calibrated P125 and Alpha 2
pumps (E.I. duPont de Nemours & Co., Wilmington, DE) were
used. Pump flow rates were approximately 0.1 L/min and sampling
time was 120 min. Sample concentrations were approximately 0.5, 1,
and 2 times the OSHA TWA-PEL for 12-L
air samples. For samples taken near the STEL (
5.8 ppm SO2 was used), a 0.1
L/min sampling rate for 15 min was used. For low concentration
samples, a test atmosphere of approximately 0.3 ppm was used.
4.2.2. Results: The results for TWA, STEL, and low
concentration-type exposures are shown in Tables 2
and 3a-3b,
respectively. The test atmosphere sample (Table
2) and spiked sample (Table
1) results for TWA samples passed the Bartlett's test and were
pooled to determine a total CV (CVT) for
the sampling and analytical method. For the experiments, the pooled
coefficients of variation, bias, and OE are as follows:
|
TWA |
STEL |
LOW |
CV |
0.048* |
0.028** |
0.032** |
Bias |
-3.3% |
+0.6% |
-6.5% |
OE |
±12.9% |
±6.2% |
±12.9% |
* |
CVT(pooled), see reference 5.11.
for further details. The CV1 is
taken from 0.5, 1, and 2 × PEL results. The 6
× PEL level is not included in statistical
calculations. |
** |
CV2
only |
4.3. Collection Efficiency
Procedure: Six commercially-prepared
(Type I) sampling tubes were used for collection at a concentration of
approximately 2 times the OSHA TWA-PEL for 120 min at 0.1
L/min (50% RH and 25 °C). The amounts of SO2
vapor collected in the first section (100 mg of sorbent) and second
section (50 mg of sorbent) were determined. The collection efficiency
(CE) was calculated by dividing the amount of
SO2 collected in the first section by the
total amount of SO2 collected in the first
and second sections.
Results: The results in Table
4 show a CE of 100%. No SO2 was found in
the second sorbent section for the CE experiment.
4.4. Breakthrough Study
(Note: Breakthrough is defined as >5% loss of analyte
through the sampling media at 50% RH)
Procedure: Two separate experiments were conducted to
test for breakthrough. For the first experiment, the same procedure as
the CE test was used with two exceptions:
- The concentration was increased to a level approximately 4 times
the TWA-PEL (7.20 ppm SO2).
- Samples were collected at 0.1 L/min for 240 min.
The second experiment was conducted using the Type II sampling tube
(Section
2.1.2.) at a concentration larger than expected during routine
sampling of industrial hygiene operations (14.8 ppm
SO2). Experimental parameters for this test
were: Four Type II sampling tubes, 25 °C, 50% RH, 240-min
sampling time, 0.1 L/min sample rate, and 14.8 ppm
SO2 test concentration.
The amount of breakthrough for each sampling tube was calculated by
dividing the amount collected in the second section by the total
amount of SO2 collected in the first and
second sections.
Results: No breakthrough of
SO2 into the second section was found.
Results for both concentrations are shown in Table
5.
4.5. Storage Stability
Procedure: A study was conducted to assess the
storage stability at 20 to 25 °C of the sampling media after
SO2 collection. Twenty-four
samples were taken near the OSHA TWA-PEL of 2 ppm. After
collection, all samples were stored under normal laboratory conditions
(20 to 25 °C) on a lab bench and were not protected from light. Six
samples were initially desorbed and analyzed, then six samples were
desorbed and analyzed after various periods of storage (6, 15, and 32
days).
Results: The mean of samples analyzed after 32 days
was within 10% of the theoretical value as shown in Table
6. A slight increase in recovery over time was noted as shown in
the figure below.
4.6. Humidity Study
Note: Prior to the validation, preliminary humidity tests
using a beaded carbon impregnated with a 10% (w/w) load of a metal
hydroxide base indicated acceptable SO2
collection at 25 and 50% RH (assuming 25 °C). Although data was also
acceptable at 80% RH (2-h sampling time), a slurry
developed inside the sampling tube. The resulting slurry presented
some difficulty in removing the bead from the sampling tube and
required an enhanced attention to technique. The slurry appeared to be
a combined result of the following factors:
- the hydrophilic nature of the impregnated base,
- the hydrophobicity of the beaded carbon, and
- complications arising from limited temperature control during
sample generations at 80% RH.
Factor (1) appeared to be the main factor in producing a slurry.
The "wetting" effect apparently was assisted by differences in
temperature during generation. The high humidity tests were conducted
during the winter season and the ambient temperature in the laboratory
was about 18 °C. The ambient temperature is normally near the test
atmosphere temperature of 25 °C; due to a malfunctioning room
thermostat it was somewhat cooler during these tests. The temperature
of the sampling tubes are ambient because of the sampling manifold
design. The test atmosphere condensation inside the tube probably was
accelerated when entering the cooler sampling tube.
The amount of base impregnation was lowered to 1% (w/w) to
alleviate potential formation of a slurry.
Procedure: A study was conducted to determine any
effect on results when samples are collected in different humidities.
Samples were taken using the generation system and procedure described
in Section
4.2. Test atmospheres were generated at 25 °C and at approximately
0.5, 1, and 2 times the OSHA TWA-PEL. Relative humidities
of 30%, 50%, and 80% were used at each concentration level tested.
Results: Results of the humidity tests are listed in
Table
7. An F test was used to determine if any significant effect
occurred when sampling at different humidities. As shown, the
calculated F values exceeded critical F values (5.14.)
for all the concentrations tested and a significant difference in
results occurred across the humidity ranges tested. An examination of
the data indicates a decrease in recoveries as humidity increases;
however, a correction for humidity effect was not instituted because
results at higher humidities were considered acceptable in terms of
overall error (< ±25%).
The finding that increasing humidity produces a decrease in the
amount of sulfate found appears to contradict previous findings in the
literature (5.15.).
The previous study (5.15.)
indicated the conversion of SO2 to sulfate
on an active carbon surface was facilitated by the presence of an
aqueous environment; however, testing carbons impregnated with a metal
hydroxide base was not performed. The base appears to significantly
alter the adsorption characteristics of the beaded carbon for
SO2.
4.7. Qualitative and Quantitative Detection Limit Study
Procedure: Low concentration samples were prepared by
spiking desorbing solutions (Section
3.3.4.) with aliquots of aqueous standards prepared from sodium
sulfate. These samples were analyzed using a 50-µL
sample injection loop and a detector setting of 1 microsiemens
(µS). A derivation of the International Union of Pure and
Applied Chemistry (IUPAC) detection limit equation (5.16.)
was used for this study.
Results: The results are shown in Table
8 for qualitative and quantitative detection limits, respectively.
The qualitative limit is 0.0187 µg/mL as
SO42- at the
99.86% confidence level. The quantitative limit (99.99% confidence) is
0.0642 µg/mL as
SO42-. Using a
12-L air volume and a 10-mL sample solution
volume, the qualitative limit is 0.004 ppm and the quantitative limit
is 0.013 ppm as SO2.
4.8. Comparison of Methods
Procedure: In order to compare the performance of
this method and to confirm the theoretical
SO2 concentrations, an independent method
(OSHA Method no. ID-104
for SO2, modified) was used. The collection
solution for Method ID-104 was modified as previously
mentioned in Section
4, and impingers were used to collect samples. The IABC and
impinger samples were collected side-by-side from the
generation system. All samples were analyzed by IC.
Results: Table
9 shows the results for different SO2
concentrations. As shown, the theoretical concentration of the
generation system, the IABC, and impinger results are in good
agreement.
4.9. Type II Tube Study
Procedure: Eight Type II sampling tubes (see Section
2.1.2. for a description of this tube) were chosen for a
statistical study using the generation system described in Section
4.2. These tubes contained PTFE membranes as prefilters. Samples
were collected side-by-side with impinger samples at 25
°C, 80% RH, and at approximately the OSHA TWA-PEL for 120
min. The high humidity was selected as a worst-case test
(Section
4.6.).
Results: Results are listed in Table
10. As shown, the Type II sampling device can be used to collect
SO2 without significantly altering the
concentration. A slight increase in recovery was noted when compared
to the earlier Type I tube results at 80% RH (Section
4.6.).
4.10. Prefilter Evaluation
Procedure: Past research regarding aerosols (5.17.)
has indicated that particulate in the air sampled may penetrate any
glass wool plugs and deposit on the sorbent when using conventional
sampling tubes. To remedy this, a prefilter is generally used to stop
the particulate before entry into the sampling tube. A preliminary
experiment was conducted using the Type II sampling tube with glass
fiber prefilters instead of PTFE. Significant losses were noted and
were apparently caused by the slightly basic glass fiber filters
reacting with some of the SO2.
To further evaluate the possibility of
SO2 reacting with a prefilter/cassette
sampling device, an experiment was performed using six Type I sampling
tubes with prefilter sampling assemblies consisting of PTFE
filter/polypropylene backup pad/carbon-filled cassettes.
These cassettes (as specified in Section
2.1.5.) have enjoyed popularity for asbestos sampling (5.18.)
and for their limited reactivity to certain corrosive gases (5.19.).
The test was conducted by taking six IABC samples without
prefilters side-by-side with six IABC with prefilters.
Samples were taken such that the test atmosphere entered the prefilter
assembly first and then entered the IABC with minimal contact at the
connection between cassette and sampling tubes. Small pieces of
Tygon® tubing were used to connect the cassettes and IABC
sampling tubes. All samples were taken at a flow rate of about 0.1
L/min for 120 min. The generation system concentration was
approximately the TWA-PEL.
Results: The results of the comparison of IABC
samples taken with and without prefilters is shown in Table
11. As shown, a difference in the amount of
SO2 collected was not noted between the
prefilter/IABC and IABC sampling assembly. The PTFE prefilter/cassette
assembly does not appear to inhibit the collection of
SO2 when using the stated sampling
conditions.
4.11. Summary
The validation results indicate the method meets both the NIOSH and
OSHA criteria for accuracy and precision (5.10.,
5.11.).
Collection efficiency, breakthrough, and storage stability are
adequate. Although it appears that humidity effects are significant
when sampling at different humidities, the results are within an
acceptable range (OE < ±25%). Detection limits are adequate when
samples are taken for 120 min at 0.1 L/min, or for 15-min
STEL determinations. The method is adequate for monitoring TWA, STEL,
and indoor air types of exposures.
The contaminant levels of blank IABC samples, when compared to
levels found in the sorbent used in OSHA Method no.
ID-107, were significantly lower. Blanks from IABC
sorbent contained only 1 to 2 µg background (as
SO42-), based on a
sample solution volume of 10 mL. The previous impregnated charcoal
sorbent contamination ranged from 20 to 100 µg
SO42-.
5. References
5.1. Occupational Safety and Health
Administration Salt Lake Technical Center: Sulfur Dioxide in
Workplace Atmospheres (Bubbler) (USDOL/OSHA Method No.
ID-104.) In OSHA Analytical Methods Manual 2nd ed.
Cincinnati, OH: American Conference of Governmental Industrial
Hygienists, 1991.
5.2. Smith, D.L., W.S. Kim, and R.E.
Kupel: Determination of Sulfur Dioxide by Adsorption on A Solid
Sorbent Followed by Ion Chromatography Analysis. Am. Ind. Hyg.
Assoc. J. 41:485-488 (1980).
5.3. Occupational Safety and Health
Administration Analytical Laboratory: Sulfur Dioxide in
Workplace Atmospheres (Solid Sorbent) (USDOL/OSHA Method No.
ID-107). In OSHA Analytical Methods Manual 1st ed.
Cincinnati, OH: American Conference of Governmental Industrial
Hygienists (Pub. No. ISBN: 0-936712-66-X), 1985.
5.4. Patty, F.A.; Ed. Industrial
Hygiene and Toxicology, 2nd rev. ed. Vol. 2. New York:
Interscience, 1963.
5.5. National Institute for Occupational
Safety and Health: Criteria for a Recommended Standard -
Occupational Exposure to Sulfur Dioxide [DHEW (NIOSH) Publication
No. 74-111]. Washington, D.C.: U.S. Government Printing
Office, 1974.
5.6. "Sulfur Dioxide" Federal Register
54:12 (19 Jan. 1989). pp 2524-2527
5.7. Occupational Safety and Health
Administration Salt Lake Technical Center: Phosphine in Workplace
Atmospheres (and Backup Data Report)(USDOL/OSHA Method No.
ID-180). In OSHA Analytical Methods Manual 2nd ed.
Cincinnati, OH: American Conference of Governmental Industrial
Hygienists, 1991.
5.8. Occupational Safety and Health
Administration Salt Lake Technical Center: Ion Chromatography
Standard Operating Procedure (Ion Chromatographic Committee). Salt
Lake City, UT. In progress.
5.9. Mandel, J.: Accuracy and Precision,
Evaluation and Interpretation of Analytical Results, The Treatment of
Outliers. In Treatise On Analytical Chemistry, 2nd ed., Vol.1,
edited by I. M. Kolthoff and P. J. Elving. New York: John Wiley and
Sons, 1978. pp. 282-285.
5.10. National Institute for Occupational
Safety and Health: Documentation of the NIOSH Validation
Tests by D. Taylor, R. Kupel, and J. Bryant (DHEW/NIOSH Pub. No.
77-185). Cincinnati, OH: National Institute for
Occupational Safety and Health, 1977. pp. 1-12.
5.11. Occupational Safety and Health
Administration Analytical Laboratory: Precision and Accuracy
Data Protocol for Laboratory Validations. In OSHA Analytical Methods
Manual 1st ed. Cincinnati, OH: American Conference of Governmental
Industrial Hygienists (Pub. No. ISBN: 0-936712-66-X),
1985.
5.12. National Institute for Occupational
Safety and Health: Backup Data Report No. S332 for Phosphine,
Attachment A. Cincinnati, OH: National Institute for Occupational
Safety and Health, 1977 (unpublished).
5.13. Davini, P.: Adsorption and
Desorption of SO2 on Active Carbon: The
Effect of Surface Basic Groups. Carbon 28:565-571
(1990).
5.14. Dowdy, S. and S. Wearden:
Statistics for Research. New York: John Wiley and Sons, 1983.
Chapter 8.
5.15. Halstead, J.A., R. Armstrong, B.
Pohlman, S. Sibley, and R. Maier: Nonaqueous Heterogeneous
Oxidation of Sulfur Dioxide. J. Phys. Chem.
94:3261-3265 (1990).
5.16. Long, G.L. and J.D. Winefordner:
Limit of Detection -- A Closer Look at the IUPAC Definition.
Anal.Chem. 55:712A-724A (1983).
5.17. Fairchild, C.I., and M.I. Tillery:
The Filtration Efficiency of Organic Vapor Sampling Tubes against
Particulates. Am. Ind. Hyg. Assoc.J. 38:277-283
(1977).
5.18. Occupational Safety and Health
Administration Salt Lake Technical Center: Asbestos (USDOL/OSHA
Method No. ID-160). In OSHA Analytical Methods Manual
2nd ed. Cincinnati, OH: American Conference of Governmental
Industrial Hygienists, 1991.
5.19. Cassenelli, M.E.: Development of a
Solid Sorbent Monitoring Method for Chlorine and Bromine in Air with
Determination by Ion Chromatography. Appl. Occup. Environ. Hyg.
6:215-226 (1991).
Table 1
Sulfur Dioxide Analysis - [Desorption Efficiency (DE)]
|
(OSHA-PEL) Taken (µg
SO2) |
Found (µg SO2) |
Recovery (F/T) |
|
N |
Mean DE |
Std Dev |
CV1 |
|
(0.5 × PEL) |
31.850 32.090 32.090 32.090 32.090 32.090 32.090 |
25.330 24.280 26.730 28.290 24.930 25.530 25.610 |
0.795 0.757 0.833 0.882 0.777 0.796 0.798 |
|
7 |
0.805 |
0.041 |
0.051 |
(1 × PEL) |
64.200 64.200 64.200 64.200 64.350 65.820 |
52.080 52.850 54.580 54.210 53.680 54.430 |
0.811 0.823 0.850 0.844 0.834 0.827 |
|
6 |
0.832 |
0.014 |
0.017 |
(2 × PEL) |
131.340 131.340 131.340 131.340 131.340 128.400 |
113.960 116.950 111.350 116.130 120.030 115.780 |
0.868 0.890 0.848 0.884 0.914 0.902 |
|
6 |
0.884 |
0.024 |
0.027 |
(6 × PEL) |
386.130 394.960 403.320 450.430 409.050 435.440 |
374.590 408.760 407.880 380.990 395.910 439.590 |
0.970 1.035 1.011 0.846* 0.968 1.010 |
|
5 |
0.999 |
0.030 |
0.030 |
* Outlier, was deleted from final statistical calculations
F/T = Found/Taken DE
= Desorption Efficiency
CV1 (Pooled) = 0.036
(Calculated from pooling 0.5, 1, and 2 × PEL data only)
The mean DEs were significantly less than 1.0; therefore, a DE
correction is needed. No corrections are necessary for
SO2 >400 µg.
|
Table 2
Sampling and Analysis - TWA Determination* (25 °C and
50% RH)
|
(OSHA-PEL) Taken (ppm
SO2) |
Found (ppm SO2) |
Recovery (F/T) |
|
N |
Mean DE |
Std Dev |
CV2 |
OE |
|
(0.5 × PEL) |
1.360 1.360 1.360 1.360 |
1.340 1.420 1.280 1.350 |
0.985 1.044 0.941 0.993 |
|
4 |
0.991 |
0.042 |
0.043 |
9.4 |
(1 × PEL) |
2.400 2.400 2.400 2.400 2.400 2.400 |
2.190 2.390 2.400 2.360 2.420 2.280 |
0.912 0.996 1.000 0.983 1.008 0.950 |
|
6 |
0.975 |
0.037 |
0.038 |
10.0 |
(2 × PEL) |
4.160 4.160 4.160 4.160 4.160 4.160 |
4.040 3.690 4.070 3.870 3.690 4.210 |
0.971 0.887 0.978 0.930 0.887 1.012 |
|
6 |
0.944 |
0.051 |
0.054 |
16.5 |
|
F/T = Found/Taken |
OE = |
Overall error (±%) |
Bias |
= |
-0.033 |
CV2 (Pooled) |
= |
0.046 |
CVT (Pooled) |
= |
0.048 |
Overall Error (Total) |
= |
12.9% |
* Samples were taken for 2
h. |
Table 3a
Sampling and Analysis - STEL Determination
(25 °C and 50% RH)
|
Sample No. |
Air Vol |
Found |
Taken |
---------------- Statistical Analysis
----------------- |
|
(L) |
(---ppm SO2---) |
N |
Mean |
Std Dev |
CV |
Recovery (%) |
|
1 2 3 4 |
1.33 1.32 1.50 1.33 |
5.26 5.35 5.56 5.22 |
5.32 5.32 5.32 5.32 |
|
4 |
5.35 |
0.15 |
0.028 |
100.6 |
|
Table 3b
Sampling and Analysis - Low Concentration
(25 °C and 50% RH) |
|
Sample No. |
Air Vol |
Found |
Taken |
---------------- Statistical Analysis
----------------- |
|
(L) |
(---ppm SO2---) |
N |
Mean |
Std Dev |
CV2 |
Recovery (%) |
|
1 2 3 4 5 6 |
12.0 12.6 11.0 12.0 12.6 11.3 |
0.288 0.288 0.300 0.288 0.300 0.275 |
0.310 0.310 0.310 0.310 0.310 0.310 |
|
6 |
0.290 |
0.009 |
0.032 |
93.5* |
|
* |
A DE correction of 0.8 was used from the scale listed
in Section
3.7.4. Low mass loadings (<30 µg) may be more
accurately corrected using the equation discussed in Section
3.7.5. |
Table 4
Collection Efficiency
(2 × TWA-PEL, 25 °C & 50% RH)
|
|
------- ppm SO2 Found
-------- |
|
Sample No. |
First Section |
Second Section |
% Collection Efficiency |
|
1 2 3 4 5 6 |
4.04 3.69 4.07 3.87 3.69 4.21 |
ND ND ND ND ND ND |
100.0 100.0 100.0 100.0 100.0 100.0 |
Notes: (a) |
Sampled at 0.1 L/min for 120 min. |
(b) |
Samples were desorbed using a sample solution volume
= 10.0 mL |
(c) |
ND = None detectable (<0.2 µg/mL as
SO42-) |
|
Table 5
Breakthrough Study
(25 °C and 50% RH)
|
|
------- ppm SO2 Found
-------- |
|
Sample No. |
First Section |
Second Section |
% Breakthrough |
|
1 2 3 4 5 6 7 8 |
7.28 6.09 6.97
7.27 15.08 15.10 14.28 13.64 |
ND ND ND ND ND ND ND ND |
0 0 0 0 0 0 0 0 |
Notes: (a) |
Sampled at 0.1 L/min for 240 min. |
(b) |
Samples were desorbed using a sample solution volume
= 10.0 mL. A 10-fold dilution of sample
solution was performed on samples 5-8. |
(c) |
ND = None detectable (<0.004 ppm
SO2) |
(d) |
Samples 1-4 used Type I sampling device;
samples 5-8 used Type II |
|
Table 6
Storage Stability Test - SO2
(1 × TWA-PEL, 25 °C, and 50% RH)
|
Day |
Air Vol |
Found |
Taken |
---------------- Statistical Analysis
----------------- |
|
(L) |
(---ppm SO2---) |
N |
Mean |
Std Dev |
CV |
Recovery (%) |
|
0 |
11.5 10.6 10.7 11.5 10.6 11.5 |
2.19 2.39 2.40 2.36 2.42 2.28 |
2.40 2.40 2.40 2.40 2.40 2.40 |
|
6 |
2.34 |
0.088 |
0.038 |
97.5 |
6 |
11.9 10.5 10.6 11.7 10.3 10.4 |
2.21 2.44 2.26 2.33 2.43 2.34 |
2.39 2.39 2.39 2.39 2.39 2.39 |
|
6 |
2.34 |
0.091 |
0.039 |
97.9 |
15 |
11.8 10.5 10.6 11.8 10.5 10.6 |
2.34 2.64 2.32 2.31 2.51 2.43 |
2.35 2.35 2.35 2.35 2.35 2.35 |
|
6 |
2.43 |
0.130 |
0.054 |
103 |
32* |
8.2 7.9 8.0 8.2 7.9 8.0 |
2.62 2.46 2.52 2.58 2.56 2.45 |
2.35 2.35 2.35 2.35 2.35 2.35 |
|
6 |
2.53 |
0.068 |
0.027 |
108 |
* |
90-min sampling time was used for this test only. The rest of
the samples were taken for 120 min. |
Table 7
Humidity Test - SO2
(0.5 × TWA-PEL & 25 °C)
|
% RH
|
30
|
50
|
80
|
|
ppm SO2 Taken
|
0.990 |
1.48 |
1.19 |
ppm SO2 Found |
1.08 0.994 0.998 1.13 1.05 1.11
|
1.33 1.41 1.27 1.35 |
1.02 1.04 1.05 1.05 1.10 1.02 |
N Mean (ppm) Std Dev (ppm) CV Ave Recovery |
6 1.06 0.057 0.054 107% |
4 1.34 0.058 0.043 90.5% |
6 1.05 0.029 0.028 88.0% |
|
At the 99% confidence level:
|
Fcrit =
6.70 |
Fcalc
= 33.81 (2, 13 degrees of freedom)
|
Fcrit <
Fcalc; therefore, a significant
difference in results was noted across the humidity levels
tested. |
Table 7 (Continued)
Humidity Test - SO2
(1 × TWA-PEL & 25 °C)
|
% RH
|
30
|
50
|
80
|
|
ppm SO2 Taken
|
2.33 |
2.40 |
2.48 |
ppm SO2 Found |
2.44 2.50 2.48 2.48 2.32 2.51
|
2.19 2.39 2.40 2.36 2.42 2.28 |
2.25 2.26 2.20 2.40 2.19 2.36 |
N Mean (ppm) Std Dev (ppm) CV Ave Recovery |
6 2.46 0.070 0.029 105% |
6 2.34 0.088 0.038 97.5% |
6 2.28 0.085 0.038 91.8% |
|
At the 99% confidence level:
|
Fcrit =
6.36 |
Fcalc
= 24.22 (2, 15 degrees of freedom)
|
Fcrit <
Fcalc; therefore, a significant
difference in results was noted across the humidity levels
tested. |
Table 7 (Continued)
Humidity Test - SO2
(2 × TWA-PEL & 25 °C)
|
% RH
|
30
|
50
|
80
|
|
ppm SO2 Taken
|
4.44 |
4.40 |
4.40 |
ppm SO2 Found |
4.28 4.32 4.25 4.41 4.72 4.59
|
4.04 3.69 4.07 3.87 3.57 4.21 |
4.03 3.67 3.94 3.81 3.96 |
N Mean (ppm) Std Dev (ppm) CV Ave Recovery |
6 4.43 0.188 0.042 99.7% |
6 3.91 0.244 0.062 88.8% |
6 3.88 0.143 0.037 88.2% |
|
At the 99% confidence level:
|
Fcrit =
6.52 |
Fcalc
= 11.88 (2, 14 degrees of freedom)
|
Fcrit <
Fcalc; therefore, a significant
difference in results was noted across the humidity levels
tested. |
Table 8
Qualitative and Quantitative Detection Limits (IUPAC
Method)
|
|
-----------
SO2 (as
SO42-) Level
-------------- |
Sample No. |
Rbl PA |
0.05 µg/mL PA |
0.10 µg/mL PA |
|
1 2 3 4 5 6 |
1.07 1.14 0.87 1.24 1.25 1.21
|
6.32 5.91 6.39 5.98 5.81 6.38 |
13.66 13.04 13.19 13.32 13.35 13.96 |
N Mean Std Dev CV
|
6 1.13 0.14 0.128 |
6 6.13 0.26 0.042 |
6 13.42 0.34
0.025 |
PA = Integrated Peak Area
(SO42-) /
1,000,000 Rbl = Reagent Blank |
|
Using the equation:
Cld = k(sd) / m
Where:
Cld |
= |
the smallest reliable detectable concentration an analytical
instrument can determine at a given confidence level. |
k |
= =
| 3 (Qualitative Detection Limit, 99.86%
Confidence) 10 (Quantitative Detection Limit, 99.99%
Confidence) |
sd |
= |
standard deviation of the reagent blank (Rbl)
readings. |
m |
= |
analytical sensitivity or slope as calculated by linear
regression. |
Cld |
= |
3(0.14) / 22.45 = 0.0187 µg/mL as
SO42- for
the qualitative limit. |
Cld |
= |
10(0.14) / 22.45 = 0.0624 µg/mL as
SO42- for
the quantitative limit. |
Qualitative detection limit |
= |
0.187 µg
SO42-
(10-mL sample volume) or 0.004 ppm
SO2 (12-L air
volume). |
Quantitative detection limit |
= |
0.624 µg
SO42-
(10-mL sample volume) or 0.013 ppm
SO2 (12-L air
volume). |
Table 9
Summary - Comparison of Methods for SO2
(50% RH & 25 °C)
|
Set#
|
Method
|
SO2 Concn (ppm)
|
N
|
Std Dev
|
|
1 |
THE 104M IABC
|
1.36 1.36 1.35 |
6 4 |
0.156 0.057 |
2 |
THE 104M IABC
|
2.40 2.31 2.34 |
6 6 |
0.058 0.058 |
3 |
THE 104M IABC
|
4.40 4.16 3.93 |
5 6 |
0.358 0.214 |
4 |
THE 104M IABC
|
5.32 5.79 5.35 |
6 4 |
0.497 0.152 |
Notes: (a) |
THE = |
Theoretical (Taken) value, calculated from certified
SO2 cylinder and gas generation system
flows. |
(b) |
104M = |
Impinger samples taken using OSHA Method No.
ID-104 (modified). |
(c) |
IABC = |
Impregnated activated beaded
carbon |
Table 10
Sampling and Analysis - Type II Sampling Tube
(1 × TWA-PEL, 25 °C, and 80% RH)
|
Sample No. |
Air Vol |
Found |
Taken |
---------------- Statistical Analysis
----------------- |
|
(L) |
(---ppm SO2---) |
N |
Mean |
Std Dev |
CV |
Recovery (%) |
|
1 2 3 4 5 6 7 8 |
10.6 10.3 11.3 12.7 10.6 10.3 11.3 12.7 |
1.65* 2.08 2.23 2.16 1.91 2.14 1.98 2.10 |
2.23 2.23 2.23 2.23 2.23 2.23 2.23 2.23 |
|
7 |
2.09 |
0.11 |
0.052 |
93.6 |
* Outlier, not used in statistical
analysis |
Table 11
Comparison Study - With/Without Pre-filter
(1 × PEL, 25 °C, and 50% RH)
Sample Set # |
With Pre-filter
|
|
Without Pre-filter
|
Air Vol, L |
ppm SO2 Found |
Air Vol, L |
ppm SO2 Found |
|
1 2 3 4 5 6 |
12.1 12.1 11.2 11.8 11.1 12.3
|
1.80 1.62 2.04 1.92 1.79 1.89 |
|
11.8 11.1 12.3 * 12.1 11.2 |
1.79 1.71 1.96 1.84 1.95 |
N Mean Std Dev CV |
6 1.84 0.14 0.077 |
|
5 1.85 0.11 0.058 |
|
* Pump stopped during sampling
Notes: (a) |
Sampling Time = 120 min |
(b) |
Flow Rate 0.1 L/min |
(c) |
Sample Solution Volume for Desorption = 10
mL |
Block Diagram of the Laboratory Generation
System
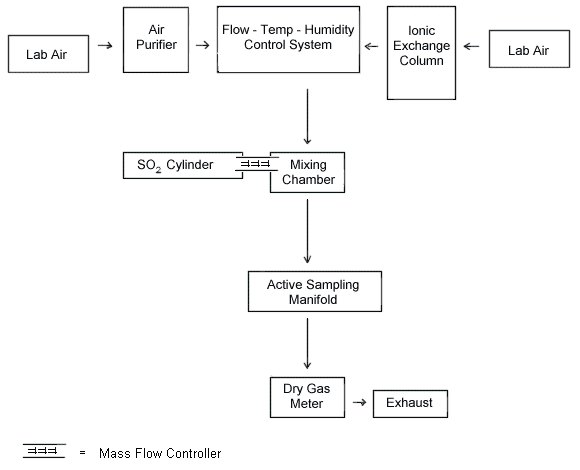
The system shown above provided a means for generating dynamic test
atmospheres. The system consists of four essential elements:
- a flow-temperature-humidity control system,
- an SO2 vapor generating system,
- a mixing chamber, and
- an active sampling manifold.
Figure 1
|