|
Method no.: |
ID-182 (This method supersedes
ID-109) |
|
|
Matrix: |
Air |
|
|
OSHA Permissible Exposure
Limits Final Rule Limit:
Transitional Limit: |
1 ppm Short-Term
Exposure Limit (STEL) 5 ppm Ceiling |
|
|
Collection Device: |
Each sample is collected using a
sampling tube containing triethanolamine-impregnated
molecular sieve (TEA-IMS) and a calibrated sampling
pump. |
|
|
Recommended Sampling Rate: |
I0.20 L/min |
|
|
Recommended Air Volume: |
3.0 L (0.20 L/min for 15 min) |
|
|
Analytical Procedure: |
The sample is desorbed from the solid
sorbent using a 1.5% triethanolamine (TEA) solution. Analysis is
performed as nitrite (NO2-) by ion
chromatography. |
|
|
Detection Limit
Qualitative: Quantitative: |
0.07 ppm (3-L air
sample) 0.19 ppm (3-L air
sample) |
|
|
Precision and Accuracy
Validation Range:
CVT: Bias
Overall Error: |
2.64 to 9.45 ppm
0.034 +0.13 ±19.8% |
|
|
Method Classification: |
Validated Method |
|
|
Chemist: |
James Ku |
|
|
Date (Date Revised): |
December 1987 (May, 1991) |
|
|
Commercial manufacturers and products mentioned in this method are
for descriptive use only and do not constitute endorsements by
USDOL-OSHA. Similar products from other sources can
be substituted.
|
|
|
Branch of Inorganic Methods Development OSHA Technical
Center Sandy City, Utah
|
1.
Introduction
This method
describes the collection and analysis of airborne nitrogen dioxide
(NO2). Samples are taken in the breathing zone of
workplace personnel and analysis is performed by ion chromatography
(IC).
1.1. History
Previous methods of analysis for
NO2 involved collection of nitrogen dioxide in bubblers
of triethanolamine (TEA) solution or a
triethanolamine-impregnated molecular sieve
(TEA-IMS) solid sorbent and TEA extraction (8.1.).
Nitrogen dioxide exposure was determined colorimetrically by the
Griess-Saltzman reaction (8.1.-8.3.).
This method, like most colorimetric procedures, can have
significant interferences. A differential pulse polarographic
(DPP) method (8.4.)
was later developed to improve sensitivity and decrease the
potential for interferences. The sensitivity of the DPP method was
adequate for measuring workplace concentrations of nitrogen
dioxide; however, the nitrite ion is unstable at the pH range (pH
1-2) used during analysis (8.5.).
Method no. ID-182 uses the collection
principle of the TEA-IMS tube. The samples are
analyzed by IC to determine NO2 exposure.
1.2.
Principle
A known volume of air is drawn through a
sampling tube containing TEA-IMS. Nitrogen dioxide is
trapped and converted to nitrite in the presence of TEA and water.
Samples are desorbed using an aqueous TEA solution and analyzed as
nitrite. The conversion mechanism of NO2 gas to nitrite
ion has been proposed by Gold (8.6.).
The following is Gold's proposal for the reaction of equivalent
amounts of NO2 and TEA in an aqueous solution:
2NO2
< = > N2O4
N2O4 +
(HOCH2CH2)3N
-->
(HOCH2CH2)3NNO+NO3-
(HOCH2CH2)3NNO+NO3-
+ H2O -->
(HOCH2CH2)3NH+NO3-
+ HNO2
HNO2 --> H+
+ NO2-
|
Nitrogen
dioxide disproportionates to nitrite and nitrate ions in the
presence of TEA. The nitrite ion (NO2-)
formed from the above reaction can be analyzed via conventional
analytical methods (8.1.-8.5.)
including IC (8.7.).
The high background levels of nitrate found in commercial
TEA-IMS sorbents ruled out further research to assess
this NO2-TEA disproportionation product by
IC.
This reaction path requires a stoichiometric factor of
0.5 for the conversion of gaseous NO2 to
NO2-. Experiments indicate the proposed
factor of 0.5 is seen only when NO2 concentrations are
greater than 10 ppm (8.6.,
8.8.-8.9.).
The conversion factor has been experimentally determined to
average approximately 0.6 to 0.7 when concentrations are below 10
ppm (8.1.-8.4.,
8.6.-8.9.).
The deviation from ideal stoichiometry is believed to be due to
other competing reactions; however, evidence to support this has
not been found (8.6.).
1.3. Advantages and Disadvantages
1.3.1. The analysis is simple, rapid, easily
automated, and specific for the nitrite ion.
1.3.2.
After sample preparation, nitrogen dioxide (as nitrite ion) can
also be determined by polarographic or colorimetric analytical
techniques (8.1.-8.4.).
1.3.3. Nitric oxide (NO) can also be sampled when using
a three-tube sampling device (8.10.).
Sulfur dioxide may also be screened using the
TEA-IMS sampling tube and similar analytical
conditions (8.7.).
1.3.4. A disadvantage is the potential interference from
large amounts of soluble chloride salts present in commercial
molecular sieve. Prior to TEA impregnation, the molecular sieve
should be washed with deionized water to remove any soluble
chloride salts.
1.3.5. Another disadvantage is the need
for a concentration-dependent conversion factor
when calculating results. 1.4. Physical Properties (8.11.)
Nitrogen dioxide (CAS No. 10102-44-0), one of
several oxides of nitrogen, is a reddish-brown or dark orange gas
with a formula weight of 46.01. Its dimer, nitrogen tetroxide
(N2O4), is colorless. At temperatures
between -9.3 and 135 °C, NO2 and
N2O4 coexist as a mixture of gases. Below
-9.3 °C, a colorless solid consisting of
N2O4 is formed, while above 135 °C, the gas
is mainly composed of NO2. Physical characteristics of
NO2 are:
Formula weight |
46.01 |
Specific gravity |
1.448 at 20 °C (liquid) |
Melting point |
-9.3 °C |
Boiling point |
21.15 °C |
Vapor pressure |
96 KPa (720 mmHg) at 20 °C |
Vapor density |
1.58 (air = 1) |
Other characteristics |
strong oxidizer, corrosive,
nonflammable |
Synonyms |
dinitrogen tetroxide, nitrogen tetroxide,
nitrogen peroxide, liquid
dioxide |
1.5.
Some sources for potential nitrogen dioxide exposures are:
agricultural silos arc or gas welding (esp.
confined space operations) electroplating plants food and
textile bleaching jewelry manufacturing nitric acid
production nitrogen fertilizer production nitro-explosive
production pickling plants Nitrogen dioxide and nitric oxide usually exist
together in industrial settings. Nitric oxide is reactive in air
and produces NO2 according to the following equations
(8.11.):
2NO
+ O2 --->
2NO2
d(NO2) / dt =
K(O2)(NO)2
|
(K is a
temperature dependent constant. At 20 °C, K = 14.8 ×
109)
An experimental approximation of the NO /
NO2 distribution found in various industrial operations
is shown (8.11.).
Source
|
% NO2
|
% NO
|
Carbon arc |
9 |
91 |
Oxyacetylene torch |
8 |
92 |
Cellulose nitrate combustion |
19 |
81 |
Diesel exhaust |
35 |
65 |
Dynamite blast |
52 |
48 |
Acid dipping |
78 |
22 |
The potential for exposure to both NO2
and NO should be considered because NO is easily oxidized to
NO2 and both oxides are likely to coexist in industrial
settings.
1.6. Toxicology
Information listed within this section is a synopsis of
current knowledge of the physiological effects of nitrogen dioxide
and is not intended to be used as a basis for OSHA policy.
1.6.1. Nitrogen dioxide is classified as a
respiratory irritant and the route of exposure is mainly
inhalation. The term silo-fillers' disease is
associated with exposure to nitrogen dioxide as well as other
nitrogen oxides.
Unlike the more soluble gases (e.g.
chlorine, ammonia) that produce almost immediate upper
respiratory tract irritation, symptoms of NO2
exposure may be delayed for up to 12 hours. The lower solubility
of NO2 provides less warning and increases the
potential for physiological damage when exposures occur.
1.6.2. The symptoms from mild exposures (<50 ppm) are
generalized below (8.12.-
8.14.):
mucoid or frothy sputum production |
cough |
painful breathing |
fever |
chest pains |
tachycardia |
increased breathing rate |
lymphocytosis |
Exposures usually result in an increased
susceptibility to respiratory infections. Changes in pulmonary
function are evident when healthy subjects are exposed to 2 to 3
ppm NO2 and can occur at far lower concentrations in
asthmatic subjects.
More severe exposures (>50 ppm)
are characterized by pulmonary edema, cyanosis, bronchiolitis
obliterans, respiratory failure and death.
1.6.3. The
LC50 (Lethal Concentration 50) for a
4-hour exposure is approximately 90 ppm
NO2. 2. Range, Detection Limit and
Sensitivity (8.8.)
2.1. This method was evaluated over the concentration
range of 2.64 to 9.45 ppm. An air volume of 3 L and a flow rate of
0.2 L/min were used. Samples were taken for 15 min. Sample results
were calculated using an average conversion relationship of:
At NO2 concentrations above 10 ppm, the
conversion factor has been shown to decrease, approaching a value
of 0.5 (8.6.,
8.8.-
8.9.).
2.2. The qualitative detection limit was 0.08 µg/mL or
0.24 µg (as NO2-) when using a
3-mL solution volume. This corresponds to 0.07 ppm
NO2 for a 3-L air volume.
2.3. The
quantitative detection limit was 0.23 µg/mL or 0.69 µg (as
NO2-) when using a 3-mL
solution volume. This corresponds to 0.19 ppm NO2 for a
3-L air volume. A 50-L sample loop and a
detector setting of 3 microsiemens were used for both detection
limit determinations.
2.4. The sensitivity of the
analytical method was calculated from the slope of a linear
working range curve (1 to 20 µg/mL nitrite). The sensitivity for
this curve was 222,720 area units per 1 µg/mL (a
Hewlett-Packard 3357 data reduction system was used,
and 1 area unit = 0.25 microvolt-second).
3. Method
Performance (8.8.)
3.1. The pooled coefficient of variation
(CVT) for samples taken in the range of 2.64 to 9.45
ppm was 0.034. The method exhibited positive bias (+0.13);
however, overall error is within acceptable limits at ±19.8%.
3.2. The collection efficiency at approximately 2 times
the PEL was 97.3%. Samples were collected at a generation
concentration of 9.45 ppm NO2 for 15 min. Sample
generation conditions were 50% RH and 25 °C.
3.3.
Breakthrough tests were performed at 30% RH and a concentration of
21 ppm. Samples were collected for 15 min at a flow rate of 0.18
L/min. Breakthrough of NO2 into a second sorbent tube
at these parameters was 1.6% NO2. This is within an
acceptable limit of <5% breakthrough.
3.4. Samples can
be stored at ambient (20 to 25 °C) laboratory conditions for a
period of at least 29 days. Storage stability results show the
mean of samples analyzed after 29 days was within ±5% of the mean
of samples analyzed after one day of storage. Samples were stored
on a laboratory bench. 4. Interferences
4.1. When other compounds are known or suspected to be
present in the sampled air, such information should be transmitted
to the laboratory with the sample.
4.2. Any compound
having the same retention time as nitrite, when using the
operating conditions described, is an interference.
4.3
Interferences may be minimized by changing the eluent
concentration, and/or pump flow rate.
4.4. If there is
reason to suspect an unresolvable interference, alternate
polarographic or colorimetric methods can be used (8.1.-8.4.).
4.5. Contaminant anions normally found in molecular sieve,
such as NO3-,
SO42-, and
PO43-, do not interfere. Large
amounts (greater than 4 to 5 µg/mL) of Cl- can
interfere. 5.
Sampling
5.1. Equipment
5.1.1. Personal sampling pumps
capable of sampling within ±5% of the recommended flow rate of
0.2 L/min are used.
5.1.2. Two types
of sampling tubes are commercially available (All molecular sieve used for tube packing
should be washed with deionized water before impregnation with
TEA):
- One type is a two-section tube packed with a
400-mg TEA-IMS front and a 200-mg
back-up section (NO2 sampling tube,
Cat. No. 226-40-02-special order, water-washed, SKC, Eighty Four, PA).
- The other type, a three-tube sampling device
(NO/NO2 sampling tubes, Cat. No.
226-40-special order, water-washed, SKC, Eighty Four, PA) can be
used to sample NO2 and NO simultaneously or
individually. The device consists of three
flame-sealed glass tubes. Nitrogen dioxide is
collected in the first tube which contains 400 mg
TEA-IMS. Two other tubes, an oxidizer tube and
another 400 mg TEA-IMS packed tube, are also
included. The dimensions of each TEA-IMS tube are
7-mm o.d., 5-mm i.d., and
70-mm long. A 3-mm portion of
silylated glass wool is placed in the front and rear of each
tube. An oxidizer tube containing approximately 1 g of a
chromate compound is used to convert NO to NO2. The
dimensions of the oxidizer tube are 7-mm o.d.,
5-mm i.d., and 110-mm long. When the
three tubes are connected in series as shown below,
NO2 and NO can be collected simultaneously.
THREE-TUBE SAMPLING DEVICE
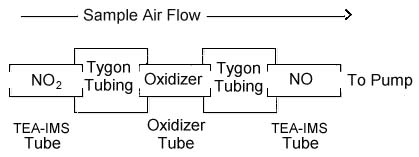 |
Text
Version: The first tube in the Three-Tube Sampling Device
is a nitrogen dioxide (NO2) sampling tube
(TEA-IMS Tube). The second tube in the series is an
oxidizer tube, and the third is another NO2
sampling tube that is identical to the first
tube. The three tubes are connected with short
lengths of plastic tubing (Tygon or equivalent). The
three tubes should be connected as close to one another as
possible. The sampling device is connected to the
sampling pump with flexible plastic tubing. The set
of three tubes that compose the sampling device is
available from SKC, Inc. as catalog 226-40.
|
For further information regarding
sampling for NO, see reference 8.10.
5.1.3. A stopwatch and bubble tube or meter are used to
calibrate pumps. A sampling tube or device is placed
in-line during flow rate calibration.
5.1.4. Various lengths of Tygon tubing are used to
connect sampling tubes to pumps. 5.2. Sampling Procedure
Note: If sampling for both NO2 and NO is necessary,
two separate pumps and sampling devices should be used. The
differences in OSHA Final Rule PELs (NO2 is a STEL and
NO is a TWA PEL) and flow rates dictates a need for a singular
assessment of NO2. Nitric oxide is collected at a flow
rate not to exceed 0.025 L/min (8.9.-8.10.)
and a three-tube device must be used. Nitrogen
dioxide can be collected at this flow rate; however, a longer
sampling time will be necessary to collect a detectable amount of
NO2 than for a short-term measurement.
Also, NO2 concentrations may vary widely during
sampling periods as long as 4 hours for NO. The
three-tube sampling device will not reflect the
varying concentration. Therefore, it is recommended to sample at
0.2 L/min for 15-min intervals using a single or two
section tube for NO2. A
separate three-tube device and pump is then used
for NO sampling. The front tube of the
device can be submitted for NO2 analysis; however,
results from this front section may not represent
short-term exposures.
5.2.1. Calibrate the sampling pumps at either
recommended flow rate listed in Section 5.2.4.
5.2.2. Connect the sampling tube or device to the pump.
The different sampling schemes are listed below:
- Sampling for NO2 only:
A single TEA-IMS tube taken from the
three-tube sampling device (Section 5.1.2.,
part b) or the two-section tube (Section 5.1.2.,
part a) can be used. If the two-section tube is
used, sampled air should enter the 400 mg section first.
- Sampling for both NO and
NO2: The three-tube device
(Section 5.1.2.,
part b) is used. Label the first tube "NO2". The
tube following the oxidizer section is labeled "NO". Also
consult reference 8.10.
5.2.3. Place the sampling tube
or device in the breathing zone of the employee.
5.2.4. Sample with pre-calibrated
pumps at the listed flow rates and sampling times:
- For NO2 only: 0.2 L/min
for at least 15 min per sample.
- For both NO and NO2:
0.025 L/min for 4 h per sample. Also consult reference 8.10.
Nitrogen dioxide results from extended sampling times
(>15 min) may not reflect short-term
exposures.
5.2.5. The minimum recommended
total air volume for collecting NO2 is 3 L.
6. Analysis
6.1. Precautions
6.1.1. Refer to instrument and standard operating
procedure (SOP) (8.15.)
manuals for proper operation.
6.1.2. Observe laboratory
safety regulations and practices.
6.1.3. Sulfuric acid
(H2SO4) can cause severe burns. Wear
protective gloves and eyewear when using concentrated
H2SO4. 6.2.
Equipment
6.2.1. Ion chromatograph (Model 2010 or 4000,
Dionex, Sunnyvale, CA) equipped with a conductivity detector.
6.2.2. Automatic sampler (Model AS-1,
Dionex) and sample vials (0.5 mL).
6.2.3. Data
processing system: Ion chromatograph interfaced to a data
reduction and control system (AutoIon 400 or 450 System,
Dionex).
6.2.4. Printer.
6.2.5. Separator and
guard columns, anion (Model HPIC-AS4A and AG4A,
Dionex).
6.2.6. Micromembrane suppressor, anion (Model
AMMS-1, Dionex).
6.2.7. Disposable syringes
(1 mL) and pre-filters.
Note: Some syringe pre-filters are not
cation- or anion-free. Tests should be
done with blank solutions first to determine suitability for the
analyte being determined.
6.2.8. Erlenmeyer flasks, 25-mL, or
scintillation vials, 20-mL.
6.2.9. Miscellaneous
volumetric glassware: Micropipettes, volumetric flasks,
graduated cylinders, and beakers.
6.2.10. Analytical
balance (0.01 mg). 6.3. Reagents -
All chemicals should be at least reagent grade.
6.3.1. Deionized water (DI H2O) with a
specific conductance of less than 10 microsiemens.
6.3.2. Triethanolamine
[(HOCH2CH2)3N]
sodium carbonate
(Na2CO3) sodium bicarbonate
(NaHCO3) sulfuric acid
(H2SO4, concentrated 95 to
98%) sodium nitrite (NaNO2)
6.3.3. Liquid desorber (1.5% TEA):
Dissolve
15 g TEA in a 1-L volumetric flask which contains
approximately 500 mL DI H2O. Add 0.5 mL
n-butanol and then dilute to volume with DI
H2O.
6.3.4. Eluent (2.0 mM
Na2CO3/1.0 mM NaHCO3):
Dissolve 0.848 g Na2CO3 and 0.336
g NaHCO3 in 4.0 L of DI H2O.
6.3.5. Regeneration solution (0.02 N
H2SO4):
Place 1.14 mL concentrated
H2SO4 into a 2-L volumetric
flask which contains about 500 mL DI H2O. Dilute to
volume with DI H2O.
6.3.6. Nitrite stock
standard (1,000 µg/mL):
Dissolve 1.5000 g
NaNO2 and dilute to the mark in a 1-L
volumetric flask with DI H2O. Prepare every 3 months.
6.3.7. Nitrite standard (100 µg/mL):
Dilute 10
mL of the 1,000 µg/mL nitrite stock standard to 100 mL with
liquid desorber. Prepare monthly.
6.3.8. Nitrite
standard (10 µg/mL):
Dilute 10 mL of the 100 µg/mL
nitrite stock standard to 100 mL with liquid desorber. Prepare
weekly.
6.3.9. Nitrite standard (1 µg/mL):
Dilute 10 mL of the 10 µg/mL nitrite stock standard to
100 mL with liquid desorber. Prepare daily.
6.4. Working
Standard Preparation
6.4.1. Nitrite working standards
(10-mL final volumes) may be prepared in the ranges specified
below:
Working Std µg/mL
|
Standard Solution µg/mL
|
Aliquot mL
|
0.5 1
3 6 10 20 |
1
1 10 10 10 100 |
5 * 3 6 * 2 |
*
Already prepared in Section 6.3. |
6.4.2. Pipette appropriate aliquots of standard
solutions (prepared in Section 6.3.)
into 10-mL volumetric flasks and dilute to volume
with liquid desorber.
6.4.3. Pipette a 0.5- to 0.6-mL
portion of each standard solution into separate automatic
sampler vials. Place a 0.5-mL filter cap into each
vial. The large exposed filter portion of the cap should face
the standard solution.
6.4.4. Prepare a reagent blank
from the liquid desorber solution. 6.5. Sample Preparation
Note: For NO sample analysis and result calculations, see
reference 8.10.
6.5.1. Clean the 25-mL Erlenmeyer
flasks or scintillation vials by rinsing with DI H2O.
6.5.2. Carefully remove the glass wool plugs from the
sample tubes, making sure that no sorbent is lost in the
process. If the two-section tube was used for
sampling, transfer each TEA-IMS section to
individual 25-mL Erlenmeyer flasks or scintillation
vials. Analyze these two sections separately. If a single
section tube was used, transfer that section to an individual
25-mL Erlenmeyer flask or scintillation vial.
6.5.3. Add 3 mL of liquid desorber to each flask or
vial, shake vigorously for about 30 s and allow the solution to
settle for at least 1 h.
6.5.4. If the sample solutions
contain suspended particulate, remove the particles using a
pre-filter and syringe. Fill the
0.5-mL automatic sampler vials with sample
solutions and push a 0.5-mL filtercap into each
vial. Label each vial.
6.5.5. Load the automatic sampler
with labeled samples, standards and blanks.
6.6. Analytical Procedure
Set up the ion chromatograph and analyze the samples in
accordance with the SOP (8.15.).
Typical operating conditions for equipment mentioned in Section 6.2.
are listed below.
Ion chromatograph
|
|
Eluent: |
2.0 mM Na2CO3 / 1.0
mM NaHCO3 |
Column temperature: |
ambient |
Sample injection loop: |
50 µL |
|
Pump
|
Pump pressure: |
approximately 1,000 psi |
Flow rate: |
2 mL/min |
|
Chromatogram
|
Run time: |
6 min |
Average retention time: |
approximately 2
min | 7. Calculations
7.1. Obtain hard copies of chromatograms from a
printer. A typical chromatogram is shown in Figure
1.
7.2. Prepare a concentration-response
curve by plotting the concentration of the standards in µg/mL (or
µg/sample if the same solution volumes are used for samples and
standards) versus peak areas or peak heights.
7.3. Blank
correct the samples by subtracting the g/mL
NO2- found in the blank from the µg/mL
NO2- found in the samples. If a different solution
volume was used for blanks and samples, use total micrograms
NO2- to blank correct.
7.4.
Calculate the concentration of nitrogen dioxide in each air sample
in ppm. A concentration-dependent conversion factor
is used. The equation is:
ppm NO2 = |
Molar volume ×
µg/mL NO2- × Solution volume ×
Conversion
Formula weight × Air volume | .
Where: |
Molar volume |
= |
24.45 (25 °C and 760 mmHg) |
µg/mL
NO2- |
= |
blank corrected sample result |
Formula weight
(NO2) |
= |
46.01 |
Conversion |
= |
varies with
concentration |
The conversion of gaseous NO2 to
NO2- is concentration-dependent
and should be calculated using one of the equations given below:
From
0 to 10 ppm, the average relationship has
been experimentally determined to be (8.1.-
8.4.,
8.6.-8.9.):
1 µg
NO2 (gas) = 0.63 µg
NO2-
or conversely:
1
µg NO2- = 1.587 µg
NO2 (gas)
Simplifying the equation and
using a 3-mL sample volume gives:
ppm nitrogen dioxide =
|
µg/mL
NO2- × 3 mL ×
0.843
Air volume (L) | Above 10 ppm NO2
|
Above 10 ppm NO2, the expected
stoichiometric factor of 0.5 mole of nitrite to 1 mole of nitrogen
dioxide gas is seen (8.6.,
8.8.-8.9.).
Therefore, the following calculation should be used for sample
results above 10 ppm and a
3-mL sample volume:
ppm nitrogen dioxide = |
µg/mL
NO2- × 3 mL 1 ×
1.063
Air volume (L) |
7.5. Reporting Results
Report all results to
the industrial hygienist as ppm nitrogen dioxide.
8.
References
8.1. National
Institute for Occupational Safety and Health: NIOSH Manual of Analytical Methods, 2nd ed.,
Vol. 4 (HEW/NIOSH Pub. No. 78-175). Cincinnati, OH,
1978.
8.2. Saltzman,
B.E.: Colorimetric Microdetermination of Nitrogen Dioxide
in the Atmosphere. Anal. Chem. 26:1949 (1954).
8.3.
Blacker, J.H.: Triethanolamine for
Collecting Nitrogen Dioxide in the TLV Range. Am. Ind. Hyg. Assoc. J. 34:390 (1973).
8.4.
Occupational Safety and Health Administration
Analytical Laboratory: OSHA Analytical
Methods Manual (USDOL/OSHA-SLCAL Method No.
ID-109). Cincinnati, OH: American Conference of
Governmental Industrial Hygienists (Pub. No. ISBN:
0-936712-66-X), 1985.
8.5.
Chang, S.K., R. Kozenianskas and G.W.
Harrington: Determination of Nitrite Ion Using Differential
Pulse Polarography. Anal. Chem.
49:2272-2275 (1977).
8.6. Gold, A.:
Stoichiometry of Nitrogen Dioxide Determination in Triethanolamine
Trapping Solution. Anal. Chem.
49:1448-50 (1977).
8.7. Vinjamoori, D.V.
and Chaur-Sun Ling: Personal Monitoring Method
for Nitrogen Dioxide and Sulfur Dioxide with Solid Sorbent
Sampling and Ion Chromatographic Determination. Anal. Chem. 53:1689-1691 (1981).
8.8. Occupational Safety and
Health Administration Technical Center: Nitrogen Dioxide Backup Report
(ID-182), by J.C. Ku. Salt Lake City, UT.
Revised 1991.
8.9. Occupational Safety and Health Administration Technical
Center: Nitric Oxide Backup Data Report
(ID-190), by J.C. Ku
(USDOL/OSHA-SLTC Method No. ID-190).
Salt Lake City, UT, Revised 1991.
8.10.
Occupational Safety and Health Administration
Technical Center: Nitric Oxide in
Workplace Atmospheres, by J.C. Ku
(USDOL/OSHA-SLTC Method No. ID-190).
Salt Lake City, UT. Revised 1991.
8.11.
National Institute for Occupational Safety and
Health: Criteria for a Recommended
Standard...Occupational Exposure to Oxides of Nitrogen (Nitrogen
Dioxide and Nitric Oxide) (HEW/NIOSH Pub. No.
76-149). Cincinnati, OH, 1976.
8.12. Berkow, R. and JH. Talbott,
ed.: The Merck Manual. 13th ed.
Rahway, NJ: Merck, Sharp and Dohme Research Laboratories, 1977.
pp. 629-630.
8.13. Proctor, N.B. and J.P. Hughes: Chemical Hazards of the Workplace.
Philadelphia, PA: J.B. Lippincott Company, 1978. pp.
382-383.
8.14. American Conference of Governmental Industrial
Hygienists: Documentation of the
Threshold Limit Values and Bioloical Exposure Indices. 5th
ed. Cincinnati, OH: ACGIH, 1986. pp. 435-436.
8.15. Occupational
Safety and Health Administration Technical Center: Standard Operating Procedure-Ion
Chromatography. Salt Lake City, UT. In progress
(unpublished).
Chromatogram of a
10 µg/mL Nitrate Standard in 1.5% TEA
Solution |
PEAK NUM |
RET TIME |
PEAK NAME |
AREA |
|
1
2 3 4
5 6 |
0.90 1.15 1.50 1.80 2.13 4.18 |
chloride nitrile |
1.778e+004 1.965e+004 2.214e+004 3.476e+003 8.886e+004 3.600e+003 |
|
|
| |