Ethylene Glycol Dinitrate (EGDN) and Nitroglycerin
(NG)
Method no.: |
43 |
|
Matrix: |
Air |
|
OSHA PEL: (ceiling) |
EGDN and/or NG NG |
- 0.2 ppm (1
mg/m3) - 0.2 ppm (2
mg/m3) (skin notations
apply) | |
|
Procedure: |
Samples are collected by drawing a known volume of
air through large (100/50-mg sorbent beds) sampling tubes containing
Tenax-GC resin. The samples are desorbed with methyl
alcohol and analyzed by liquid chromatography with a Thermal Energy
Analyzer or an ultraviolet HPLC detector. |
|
Recommended air volume and sampling rate: |
15 L at 1 L/min |
|
|
|
Reliable quantitation limits: |
2.0 ppb (12
µg/m3) |
2.0 ppb (19
µg/m3) | |
|
Standard error of estimate at the OSHA
PEL: (Section 4.7.) |
6.9 % |
8.1 % |
|
ppb = parts per
billion | |
|
Special requirement: |
The sampling pump must be certified by NIOSH and/or
MSHA (formerly MESA) as intrinsically safe for use in coal
mines. |
|
Status of method: |
A sampling and analytical method that has been
subjected to the established evaluation procedures of the Organic
Methods Evaluation Branch. |
|
Date: February 1983 |
Chemist: Warren
Hendricks |
Organic Methods Evaluation Branch OSHA Analytical
Laboratory Salt Lake City, Utah
1. General discussion
1.1. Background
1.1.1. History
The purpose of this work was to evaluate air sampling and
analytical procedures which permit the simultaneous determination of
EGDN and NG. It is necessary to determine the analytes together
because the OSHA standard regulates exposure to EGDN and/or
NG.
Previously, air samples for EGDN and NG have been collected with
midget impingers containing ethanol (Ref. 5.1.), and with sampling
tubes containing silica gel (Ref. 5.1.), Chromosorb 102 porous
polymer beads (Ref. 5.2.), or Tenax-GC resin (Ref.
5.3.). EGDN and NG have been determined by colorimetry (Ref. 5.1.)
and by gas chromatography (GC) using an electron capture detector
(Ref. 5.3.). EGDN and NG have also been analyzed by high performance
liquid chromatography (HPLC) using various means of detection
including electrochemical (Ref. 5.4.), photoconductivity (Ref. 5.5),
electron capture (Ref. 5.6.), and chemiluminescence (Ref. 5.7.).
Tenax-GC resin was selected for evaluation as a
collection medium for EGDN and NG because of published
recommendations and also because initial laboratory tests indicated
that the material would perform satisfactorily. An HPLC separation
procedure was chosen over GC methods because of the inherent thermal
instability of the analytes (Ref. 5.7.). The Thermal Energy Analyzer
(TEA) detector was selected because it has been shown to have a
sensitive and selective response to the analytes (Ref. 5.7.). An
HPLC ultraviolet detector can be substituted for the TEA but this
will result in less selectivity for the analytes.
The volatile nature of EGDN has been reported in the literature
(Ref. 5.8.) and it was shown, by vapor spiking experiments (Section
4.11.), that the compound could be collected on
Tenax-GC resin with high efficiency. NG, however,
because of its low vapor pressure (Section 1.1.4.), could exist as
an aerosol. The collection efficiency of NG on Tenax-GC
resin was investigated using a Model 3050 Bergland-Liu Vibrating
Orifice Monodisperse Aerosol Generator to produce a test atmosphere
which contained an aerosol component. The presence of an aerosol was
indicated by a Model 3200 Thermo Systems Inc. Particle Mass Monitor.
Glass fiber filters, midget bubblers containing ethanol, small
(20/10-mg sorbent beds) Tenax-GC resin tubes, and large
(100/50-mg sorbent beds) Tenax-GC resin tubes were
evaluated as sampling media for NG. The aerosol test atmospheres
contained 1 mg/m3 of NG. Glass fiber
filters proved to be an ineffective sampling medium because of low
retention efficiency for NG. The small Tenax-GC resin
tubes gave slightly higher results than did the large
Tenax-GC tubes when both were compared to the ethanol
bubbler. (Section 4.10.) The large Tenax-GC tube was
selected because EGDN presented breakthrough and migration problems
on the small size Tenax-GC tubes (Section 2.4.).
Because it is more convenient to use solid sorbents than bubblers,
the Tenax-GC tubes were selected as the test sampling
medium for EGDN and NG.
1.1.2. Toxic effects (This section is for information only and
should not be taken as the basis of OSHA policy).
The OSHA PEL for EGDN and/or NG is 0.2 ppm (1
mg/m3) and the PEL for NG is 0.2 ppm (2
mg/m3). Both of these standards are for
ceiling concentrations. An EGDN footnote states that "An atmospheric
concentration of not more than 0.02 ppm or personal protection may
be necessary to avoid headache". (Ref. 5.9.)
The effects of exposure to EGDN and NG are reported to be
similar. Both compounds are potent vasodilating agents. Both
chemicals are easily absorbed by inhalation and through the skin.
The greatest exposure to workers who directly handle these compounds
is probably through skin absorption (Ref. 5.10.).
The effects of exposure to NG were first reported over 100 years
ago. The most frequently reported symptoms of exposure to EGDN or NG
include intense headaches, dizziness, nausea and decreases in
systolic, diastolic and pulse blood pressure. These symptoms are a
result of the rapid shift of blood volume from the central to the
peripheral circulatory system, caused by the dilation of the blood
vessels. After 2 to 4 days of occupational exposure to NG or EGDN,
most workers no longer experience symptoms because they have become
tolerant to the vasodilatory effects of the compounds (Ref. 5.10.).
Angina pectoris has been reported among workers who were exposed
to EGDN and/or NG. In those affected, the angina usually occurred in
periods away from work. Sudden deaths without any apparent cause
have also been reported among these workers. The deaths, like the
angina, occurred more frequently during periods away from work. In
most cases, the workers who died suddenly had no symptoms other than
angina during periods away from work. The deaths are thought to be
related to compensatory vasoconstriction (tolerance) induced by
repeated exposure to the substances. Vasoconstriction is thought to
lead to spasms of the coronary arteries and then the related angina
pectoris and sudden deaths (Ref. 5.10.).
Historically, it has been noted that wives of dynamite workers
often experienced heavy menstrual bleeding and had fewer children
than did other women. It was also reported that children of dynamite
workers were born prematurely and were cyanotic or were not as
strong as other children (Ref. 5.10.).
No evidence of teratogenic and embryotoxic effects were observed
after the intraperitoneal administration of as much as 20 mg/kg NG
to rats during gestation and lactation (Ref. 5.11.).
The potential carcinogenicity of NG has been studied in rats and
mice. In the rat study, NG was administered in a 0.03% solution as
the drinking water. The investigators concluded that NG was not
carcinogenic under the experimental conditions. The mice were
exposed to time-weighted average concentrations of 214 mg/L in their
drinking water. Increased incidence of adenomas of the pituitary
gland was found in the female mice. It was concluded that NG was not
carcinogenic under the experimental conditions because the tumors
were benign. Even though the tumors were benign, their development
indicates that exposure to NG can affect the pituitary gland (Ref.
5.10.).
1.1.3. Potential workplace exposure
EGDN and NG are used with a mixture of sodium nitrate and an
absorbent, often wood pulp, to produce dynamite. EGDN is added to
lower the freezing point of the EGDN/NG mixture and is currently the
major component. The EGDN/NG ratio is about 8/2 or 9/1. This is the
only commercial use for EGDN. Because EGDN is more volatile than NG,
there is usually more airborne EGDN than NG from the dynamite
mixture. In 1976, about 250 million pounds of dynamite, containing 5
to 50% EGDN/NG, were produced by U.S. manufacturers (Ref. 5.10.).
NG is used to make smokeless gun powder and rocket propellants.
Single-base powders contain only nitrocellulose, double-base powders
contain nitrocellulose and NG, and triple-base powders contain
nitrocellulose, NG, and other combustible materials (Ref. 5.10.).
NG is used for medical purposes, primarily to treat angina
pectoris and other circulatory disorders (Ref. 5.10.).
Therefore, occupations with potential exposure to EGDN and NG
include chemical and explosives workers, drug makers, dynamite
makers, miners, missile technicians, munitions loaders, munitions
workers, NG workers, rocket fuel makers, shell fillers and
smokeless-powder makers. NIOSH estimates that about 8,000 persons in
the United States may be exposed to EGDN/NG mixtures or to NG alone
(Ref. 5.10.).
1.1.4. Physical properties (Ref. 5.10.).
|
EGDN |
NG |
CAS no.: |
55-63-0 |
628-96-6 |
molecular weight: |
152.06 |
227.09 |
specific gravity (20°C): |
1.49 |
1.59 |
freezing point (°C): |
-22.3 |
13.3 |
vapor pressure (mm Hg at 20°C): |
0.038 to 0.05 |
0.00012 to 0.011 |
explosion point (°C): |
114 |
256 |
physical appearance: |
yellowish liquid |
pale yellow viscous
liquid |
EGDN is insoluble in water, but miscible with most organic
solvents. NG is slightly soluble in water, soluble in alcohol and
ether.
Structure: |
EGDN
 |
NG
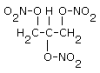 |
A labile, rotational isomer of NG exists; however, it is
converted to the stable form after 1 - 2 weeks of storage (Ref.
5.12.).
Synonyms for EGDN, NG and EGDN/NG Mixtures (This data taken
directly from Ref. 5.10.)
Ethylene Glycol Dinitrate 1,2-Ethanediol dinitrate*;
EGDN; Ethylene dinitrate; Ethylene nitrate; Glycol dinitrate;
Nitroglycol.
Nitroglycerin 1,2,3-Propanetriol trinitrate*; Angibid;
Anginine; Angiolingual; Angorin; Blasting gelatin; Blasting oil;
Cardamist; Glondin; Glycerin trinitrate; Glycerol trinitrate; GTN;
Lenitral; NG; Niglycon; Nitric acid triester of glycerol;
Nitrine-TDC; Nitro-glycerin; Nitroglycerin, liquid undesensitized**;
Nitroglycerine; Nitroglycerol; Nitroglyn; Nitrol; Nitrolan;
Nitro-lent; Nitrolingual; Nitrolowe; Nitromel; Nitrong; Nitrorectal;
Nitroretard; Nitro-Span; Nitrostat; Nitrozell retard; NTG;
Nysconitrine; Perglottal; Propanetriol trinitrate;
1,2,3-Propanetriyl nitrate; S.N.G.; Soup; Trinalgon; Trinitrin;
Trinitroglycerin; Trinitroglycerol; Vasoglyn.
EGDN/NG Mixtures Nitroglycerine; Nitroglycerol;
Nitroglycol; Nitroglyn; Nitrogranulogen;
alpha-Nitroguanidine; beta-Nitroguanidine;
2-Nitro-2-heptene; 3-Nitro-2-heptene;
3-Nitro-3-heptene; 4-Nitro-3-heptene;
2-Nitro-2-hexene.
*International Union of Pure and Applied Chemistry (IUPAC) common
name **Department of Transportation (DOT)
1.2. Limit defining parameters (by TEA detector) (The analyte air
concentrations listed throughout this method are based on an air
volume of 15 L and a desorption volume of 2.0 mL).
1.2.1. Detection limits of the analytical procedure
The detection limits of the analytical procedure for EGDN and NG
are 0.93 and 1.4 ng per injection respectively. These are the
amounts of analytes which will give peaks whose heights are about 5
times the height of the baseline noise (Section 4.1.).
1.2.2. Detection limits of the overall procedure
The detection limits of the overall procedure for EGDN and NG are
186 ng (2.0 ppb or 12 µg/m3) and 280 ng
(2.0 ppb or 19 µg/m3) per sample
respectively. These are the amounts of EGDN and NG spiked on the
sampling device which allow recoveries of the analytes approximately
equivalent to the detection limits of the analytical procedure
(Section 4.2.).
1.2.3. Reliable quantitation limits
The reliable quantitation limits for EGDN and NG are 186 ng (2.0
ppb or 12 µg/m3) and 280 ng (2.0 ppb or 19
µg/m3) per sample respectively. These are
the smallest amounts of the analytes which can be quantitated within
the requirements of a recovery of at least 75% and a precision (1.96
SD) of ±25% or better (Section 4.2.).
The reliable quantitation limit and detection limits reported in
the method are based upon optimization of the instrument for the
smallest possible amount of analyte. When the target concentration of
an analyte is exceptionally higher than these limits, they may not be
attainable at the routine operating parameters.
1.2.4. Sensitivity
The sensitivities of the analytical procedure over concentration
ranges representing 0.5 to 2 times the OSHA PEL, based on the
recommended air volume, are 16890 area units per µg/mL for EGDN and
14120 area units per µg/mL for NG. These are determined by the slope
of the calibration curves (Section 4.4.). The sensitivity will vary
with the particular instrument used in the analysis.
1.2.5. Recovery
The recoveries of the analytes from samples used in a 17-day
storage test remained above 93.1% for EGDN and 95.1% for NG when the
samples were stored at ambient temperature (Section 4.7.). The
recovery of each analyte from the collection medium after storage
must be 75% or greater.
1.2.6. Precision (analytical method only)
The pooled coefficients of variation obtained from replicate
determinations of analytical standards at 0.5, 1, and 2 times the
OSHA PEL were 0.068 for EGDN and 0.053 for NG (Section 4.3.).
1.2.7. Precision (overall procedure)
The precisions at the 95% confidence level for samples used in
the 17-day storage test were ±13.5% for EGDN and ±15.8% for NG
(Section 4.7.). These values each include an additional ±5% for
sampling error. The overall procedure must provide results at the
OSHA PEL that are ±25% or better at the 95% confidence level.
1.2.8. Reproducibility
Six vapor spiked (Section 4.11.) samples and a draft copy of this
procedure were given to a chemist unassociated with this evaluation.
The samples were analyzed after 5 days of storage at ambient
temperature. The average recoveries (corrected for desorption
efficiency) were 103% for EGDN and 94.6% for NG. The standard
deviations were 4.7% for EGDN and 6.1% for NG (Section 4.9.).
1.3. Advantages
1.3.1. The sampling and analytical procedures are precise,
reliable and convenient.
1.3.2. The air sampling device is commercially available.
1.4. Disadvantages
1.4.1. This method has not been field tested.
1.4.2. The TEA detector is quite expensive.
2. Sampling Procedure
2.1. Apparatus
2.1.1. Samples are collected by use of a personal sampling pump
that can be calibrated to within ±5% of the recommended flow rate
with the sampling device in line. The sampling pump must be
certified by NIOSH and/or MSHA (formerly MESA) as intrinsically safe
for use in coal mines.
2.1.2. Samples are collected on sampling tubes containing 35/60
mesh Tenax-GC resin. The tube has two sections of resin
separated by a glass wool plug. The front (sampling) section
contains 100 mg of resin and the back section 50 mg. The sections
are held in place by glass wool plugs in an 8-mm o.d. × 100 mm long
glass tube. SKC, Inc. Tenax-GC resin sampling tubes
(catalog no. 226-35-03) were used in this evaluation.
2.2. Reagents
None required
2.3. Technique
2.3.1. Break open both ends of the flame-sealed
Tenax-GC sampling tubes so that the holes in the tube
ends are at least one-half the i.d. of the sampling tube. Connect
the sampling tube to the sampling pump with flexible tubing. The
smaller section of the sampling tube is used as a backup and should
be positioned nearest the sampling pump. Sampled air should not pass
through any hose or tubing before entering the sampling tube.
2.3.2. Place the sampling tube vertically in the employee's
breathing zone.
2.3.3. After sampling, seal the tubes immediately with plastic
caps and wrap lengthwise with OSHA Form 21.
2.3.4. Submit at least one blank for each sample set. The blank
should be handled in the same manner as samples, except no air is
drawn through it.
2.3.5. Record sample volume (in liters of air) for each sample,
along with any potential interferences.
2.3.6. Ship any bulk sample(s) in a separate container(s) from
the air samples.
2.4. Retention efficiency
Retention efficiency studies were performed by first vapor spiking
(Section 4.11.) 74 µg of EGDN and 112 µg of NG on the recommended
sampling device. An appropriate volume of humid air was pulled through
the sampling tube and the device was analyzed. Retention efficiency
was defined as the percent of an analyte remaining on the sampling
section of the tube after air had been pulled through the device.
The retention efficiency for EGDN was found to be greater than 95%
after 426 L of air at about 80% relative humidity and 22°C had been
pulled through the vapor spiked tube. The retention efficiency for NG
was determined to be 100% on the same sample (Section 4.5.).
Because retention efficiencies for EGDN and NG remained high at
large air volumes, the smaller size (20/10-mg sorbent beds)
Tenax-GC sampling tubes were evaluated to determine
analyte breakthrough. This study was performed by connecting the
sampling sections of two tubes in series and then vapor spiking the
first section with 37 µg of EGDN and 56 µg of NG. Humid air was pulled
through the sampling train at 1 L/min, the second sample was removed
at appropriate times and then subjected to analysis. The second tube
was replaced with a fresh sampling tube and sampling continued. The
first tube was also analyzed after the study was terminated.
Two breakthrough studies were performed using the smaller tubes and
in both experiments, EGDN was observed in the second tube before 10 L
of air had been sampled. The average 5% breakthrough air volume for
EGDN was about 33 L. No breakthrough was observed for NG after 55 L
had been sampled (Section 4.5.).
About 19% of the EGDN spiked on small size Tenax-GC
resin tubes was found to migrate from the sampling to the reference
section after storage at ambient temperature for 7 days. No migration
was observed for NG after storage at ambient temperature for 14 days
(Section 4.5.).
The 5% breakthrough air volume for EGDN using the small tubes was
shown to be sufficiently high to permit sampling the recommended air
volume; however, the rapid appearance of EGDN in the reference portion
of the sampling tube indicated that the device may be subject to
channeling. The migration and channeling problems were such that the
small Tenax-GC resin tubes were eliminated from further
consideration as sampling media for EGDN. Since it was desirable to
determine EGDN and NG on the same sample, the small tubes were not
evaluated for NG alone.
2.5. Desorption efficiency
The average desorption efficiencies for EGDN and NG from samples
spiked at 0.5, 1 and 2 times the OSHA PEL, were 98.1% and 99.4%
respectively (Section 4.6.).
2.6. Recommended air volume and sampling rate
2.6.1. The recommended air volume is 15 L.
2.6.2. The recommended sampling rate is 1 L/min.
2.7. Interferences (sampling)
2.7.1. There are no known interferences to the sampling method.
2.7.2. Suspected interferences should be reported to the
laboratory with submitted samples.
2.8. Safety precautions (sampling)
2.8.1. The air sampling pump must be certified by NIOSH and/or
MSHA (formerly MESA) as intrinsically safe for use in coal mines.
2.8.2. Exercise due caution when breaking open the sampling
tubes. Take measures to prevent cuts from the sharp ends of the
broken glass tubes.
2.8.3. Attach the sampling equipment to the worker in such a
manner that it will not interfere with work performance or safety.
2.8.4. Follow all safety practices that apply to the work area
being sampled.
3. Analytical Procedure
3.1. Apparatus
3.1.1. An HPLC apparatus equipped with a TEA and/or UV detector.
For this evaluation, a Waters Extended Wavelength Module/Waters
Model 440 Absorbance Detector (214 nm) and a Thermo Electron
Corporation Model 502 TEA (EAP) were used in series with a Waters
M-6000A pump.
3.1.2. An HPLC column capable of resolving the analytes from each
other and potential interferences. The column used in this work was
a DuPont Zorbax CN, 4.6 mm × 25 cm.
3.1.3. An electronic integrator or other suitable means to
measure peak area and record chromatograms. A Hewlett-Packard 3354
B/C Data System was used in this evaluation.
3.1.4. Vials, 4-mL, with Teflon-lined caps. Waters WISP vials
were used in this evaluation.
3.1.5. Volumetric flasks, pipets and syringes for preparing
standards, making dilutions and making injections.
3.1.6. Dewar flasks, for liquid nitrogen.
3.2. Reagents
3.2.1. HPLC grade methanol, isopropanol and isooctane.
3.2.2. Technical grade n-propanol or ethanol for cold traps.
3.2.3. Liquid nitrogen.
3.2.4. GC grade helium.
3.2.5. Medical grade oxygen.
3.2.6. Standard EGDN. A solution containing 1 g EGDN in 100 mL of
ethanol was obtained from Atlas Powder Co., Tamqua, PA, for use in
this evaluation.
3.2.7. Standard NG. A solution containing 1 g NG in 100 mL of
dichloromethane was obtained from Hercules, Inc., Magna, UT, for use
in this evaluation.
3.3. Standard preparation
3.3.1. Prepare stock standards by diluting known amounts of EGDN
and NG with methanol.
3.3.2. Prepare an intermediate standard mixture using known
volumes of the stock standard and diluting the mixture with
methanol. The intermediate standard should contain 0.93 mg/mL EGDN
and 1.4 mg/mL NG.
3.3.3. Prepare fresh working range standards daily by diluting
the intermediate standard mixture with methanol. Standards
representing the OSHA PEL were obtained by diluting the intermediate
standard mixture 1 to 50 with methanol.
3.3.4. Prepare standards at concentrations other than the OSHA
PEL in order to generate the calibration curve.
3.3.5. Store the standards in a freezer using well-sealed, dark
containers.
3.4. Sample preparation
3.4.1. Transfer each section of the sample to separate vials.
Place the front glass wool plug in the vial with the front section
of the Tenax-GC tube. Discard the other glass wool
plugs.
3.4.2. Add 2.0 mL of methanol to each vial.
3.4.3. Seal the vials with Teflon-lined caps and allow them to
desorb for 1 h. Gently shake the vials several times during the
desorption time.
3.5. Analysis
3.5.1.
column: |
DuPont Zorbax CN (4.6 mm × 25 cm) |
solvent: |
isooctane/isopropanol/methanol (90:6:4)
(v/v) |
flow rate: |
1 mL/min |
injection volume: |
10 µL |
retention time: |
EGDN - 8.4 min NG - 11.5
min |
3.5.2. UV detector
3.5.3. TEA detector
HPLC pyrolyzer temp: |
550°C |
HPLC interface temp: |
100°C |
oxygen flow rate: |
5 mL/min |
helium flow rate: |
30 mL/min (carrier gas) |
cold trap temp: |
-80°C (ethanol/water or n-propanol/water,
with liquid nitrogen) |
3.5.4. Chromatograms:
Section
4.8.
3.5.5. Detector response is measured with an electronic
integrator or other suitable means.
3.5.6. Use an external standard method to prepare the calibration
curve with at least three standard solutions of different
concentrations. Prepare the calibration curve daily. program the
integrator to report results in µg/mL.
3.5.7. Bracket sample concentrations with standards.
3.6. Interferences (analytical)
3.6.1. Any compound with the same general retention time as EGDN
or NG and which also gives a detector response is a potential
interference. Possible interferences should be reported to the
laboratory with submitted samples by the industrial hygienist.
3.6.2. HPLC parameters (solvent composition, column, detector,
etc.) may be changed to possibly circumvent interferences.
3.6.3. The only unequivocal means of structure designation is by
GC/MS. It is recommended this procedure be used to confirm samples
whenever possible.
3.7. Calculations
3.7.1. Results are obtained by use of a calibration curve. The
detector response, for each standard, is plotted against its
concentration in µg/mL and the best straight line through the data
points is determined by linear regression.
3.7.2. The concentration, in µg/mL, for a particular sample is
determined by comparing its detector response to the calibration
curve. If any EGDN or NG is found on the backup section, it is added
to the amount found on the front section. This total amount is then
corrected by subtracting any interference found in the blank.
3.7.3. The EGDN and/or NG air concentration can be expressed
using the following equations:
mg/m3 EGDN or NG =
(A)(B)/(C)(D)
where |
A |
= |
µg/mL from Section 3.7.2. |
|
B |
= |
desorption volume (mL) |
|
C |
= |
sample air volume (L) |
|
D |
= |
desorption
efficiency |
or ppm = (mg/m3)(24.46)/molecular
weight
where |
molecular weights |
= |
EGDN, 152.06; NG, 227.09 |
|
24.46 |
= |
molar volume of an ideal gas at 760 mm Hg and
25°C |
3.8. Safety precautions (analytical)
3.8.1. Avoid skin contact and inhalation of all chemicals used.
3.8.2. Restrict the use of all chemicals to a fume hood whenever
possible.
3.8.3. The handling of EGDN and NG, especially in more
concentrated forms, may be hazardous because of the instability of
the compounds.
3.8.4. Check to be sure that the TEA exhaust is connected to a
fume hood.
3.8.5. Wear safety glasses and a lab coat in all laboratory
areas.
4. Backup Data
4.1. Detection limit of the analytical procedure
The detection limit of the analytical procedure was 0.93 ng for
EGDN and 1.4 ng for NG. These amounts produce peaks whose heights were
about 5 times the height of the baseline noise (Figure 4.1.).
4.2. Detection limit of the overall procedure and reliable
quantitation limit
The detection limit of the overall procedure was 186 ng/sample for
EGDN and 280 ng/sample for NG. The equivalent air concentrations were
12 µg/m3 (2.0 ppb) for EGDN and 19
µg/m3 (2.0 ppb) for NG. The 10-µL injection
size recommended in the analytical procedure was used in the
determination of the detection limit of the overall procedure.
The reliable quantitation limits were determined by liquid spiking
6 large Tenax-GC resin tubes with 186 ng of EGDN and 280
ng of NG. The samples were desorbed with 2.0 mL of methanol and
subjected to analysis. The 10 µL-injection volume recommended in the
analytical procedure was used to determine the reliable quantitation
limits.
Table 4.2. Reliable Quantitation Limit Data
|
|
% recovery |
sample no. |
EGDN |
NG |
|
1 2 3 4 5 6
 SD 1.96
SD |
85.7 100 84.9 93.7 98.0 80.3
90.4 7.9 16 |
96.3 98.4 100 100 88.6 91.0
95.7 4.8 9.5 |
|
Since the recoveries were near 100% and also the precisions were
better than ±25%, the detection limits of the overall procedure and
the reliable quantitation limits were the same.
4.3. Precision (analytical method only)
The following data were obtained from multiple injections of
analytical standards. The data are also presented graphically in
Figures 4.3.1. and 4.3.2.
Table 4.3.1. Sensitivity and Precision Data for
EGDN
|
× target conc. µg/mL |
0.5× 4.65 |
1× 9.3 |
2× 18.6 |
|
area
counts
 SD CV
= 0.068 |
68505 68152 73602 78557 75178 81354
74224.7 5300.7 0.0714 |
161111 148645 142462 139825 132371 127943
142059.5 11871.2 0.0836 |
328323 305681 318531 303060 301729 288860
307697.3 13847.9 0.0450 |
|
Table 4.3.2. Sensitivity and Precision Data for NG
|
× target conc. µg/mL |
0.5× 7.0 |
1× 14.0 |
2× 28.0 |
|
area
counts
 SD CV
= 0.053 |
135816 126207 128996 122751 125092 117101
125993.8 6260.1 0.0497 |
226043 230653 242265 244586 258245 270668
245410.0 16769.8 0.0683 |
405955 417600 419134 439648 428030 450057
426737.3 16033.5 0.0376 |
|
4.4. Sensitivity
The sensitivity for EGDN was 16890 area counts per µg/mL and that
for NG was 14120 area counts per µg/mL. These values were determined
from Figures 4.3.1. and 4.3.2.
4.5. Retention efficiency data
Retention efficiency studies were performed by vapor spiking
(Section 4.11.) 74 µg of EGDN and 112 µg of NG on each of several
large Tenax-GC resin (100/50 mg sorbent beds) tubes.
Humid air (about 80% RH and 22°C) was drawn through the tubes at 1
L/min. The tubes were then subjected to analysis and the results are
presented in Table 4.5.1.
Table 4.5.1. Retention Efficiency
|
air volume L |
EGDN recovery front sec.,% |
EGDN recovery back sec.,% |
NG recovery front sec.,% |
NG recovery back sec.,% |
|
171.3 248.1 305.0 333.0 370.0 383.0 397.0 426.0 |
100 100 100 99.6 98.6 98.5 98.1 97.0 |
ND Trace Trace 0.4 1.4 1.5 1.9 3.0 |
100 100 100 100 100 100 100 100 |
Trace ND ND ND ND ND ND ND |
|
Two breakthrough experiments were performed with small
Tenax-GC resin (20/10-mg sorbent beds) tubes. The studies
were conducted by connecting the sampling sections of two tubes in
series and then vapor spiking the first section with 37 µg of EGDN and
56 µg of NG. Humid air (at about 80% RH and 22°C) was drawn through
the sampling train at 1 L/min. The second tube was removed at
appropriate intervals and subjected to analysis. The second tube was
replaced with a fresh sampling tube and sampling continued. The first
tube was also analyzed after the study was terminated. No breakthrough
was observed for NG. The results of the two breakthrough studies are
presented in Table 4.5.2.
Table 4.5.2. Breakthrough Studies
|
study one |
study two |
|
|
air volume, L |
cumulative EGDN breakthrough,
% |
air volume, L |
cumulative EGDN breakthrough,
% |
|
4.5 13.6 22.7 31.8 40.9 50.0 |
0.7 1.7 3.1 4.3 5.1 7.8 |
9.2 18.4 27.6 36.8 46.0 55.2 |
1.4 3.2 5.3 6.3 6.9 9.2 |
|
All of the vapor spiked NG was recovered from the first tube in
both studies. The EGDN recovery for the first tube in Study One was
92.2% and that for the first tube in Study Two was 90.8%.
A migration study was performed with small Tenax-GC
tubes to determine if either analyte would move from the sampling to
the back section of the tubes. The device was liquid spiked with 19 µg
of EGDN and 28 µg of NG and then stored at ambient temperature. No
migration was observed for NG; however, considerable migration and
some loss was noted for EGDN.
Table 4.5.3. Migration Study
|
|
sampling section |
back section |
|
|
|
|
recovery, % |
recovery, % |
day |
EGDN |
NG |
EGDN |
NG |
|
0 7 14 |
100 68.2 57.6 |
96.3 99.2 93.6 |
-- 19.2 19.2 |
-- -- -- |
|
4.6. Desorption efficiency
The following data represent the analysis of large
Tenax-GC tubes liquid spiked with EGDN and NG at 0.5, 1,
and 2 times the OSHA PEL.
Table 4.6. Desorption Efficiency
|
× PEL |
0.5× |
1× |
2× |
|
|
|
|
analyte |
EGDN |
NG |
EGDN |
NG |
EGDN |
NG |
µg/sample |
9.3 |
14.0 |
18.6 |
28.0 |
37.2 |
56.0 |
|
desorption efficiencies,
%
 |
102 105 102 96.9 95.4 98.5
100 |
102 104 102 98.9 97.7 98.3
100 |
99.5 97.1 96.3 97.7 96.3 105
98.6 |
103 98.9 94.2 93.7 93.8 100
97.3 |
92.8 97.9 97.1 98.4 95.9 93.1
95.9 |
101 103 101 101 99.2 98.9
101 |
|
The average desorption efficiency for EGDN was 98.1% and that for
NG was 99.4%.
4.7. Storage data
The data in Table 4.7.1. represent the effects of storage at
ambient (21 to 26°C) and reduced (-20°C) temperature on
large Tenax-GC tubes vapor spiked (Section 4.11.) with
18.6 µg of EGDN and 28.0 µg of NG. The results are not corrected for
desorption efficiency. The data are also presented graphically in
Figures 4.7.1. - 4.7.4.
Table 4.7.1. Storage Tests for EGDN
|
storage time |
% recovery |
(days) |
(refrigerated) |
|
(ambient) |
|
0 3 7 10 14 17 |
101 97.4 91.9 94.4 91.1 106 |
101 93.9 104 93.3 89.2 95.6 |
102 92.9 98.0 95.9 96.2 101 |
|
98.0 100 96.3 96.3 87.1 98.5 |
97.3 95.4 91.0 94.8 88.4 95.8 |
94.1 99.8 95.5 92.5 94.1 95.0 |
|
Table 4.7.2. Storage Tests for NG
|
storage time |
% recovery |
(days) |
(refrigerated) |
|
(ambient) |
|
0 3 7 10 14 17 |
103 97.1 89.0 93.9 90.1 109 |
94.0 93.1 106 93.6 89.3 92.1 |
103 93.7 100 99.0 95.7 106 |
|
99.7 99.9 96.5 100 85.9 101 |
95.9 93.0 92.2 95.3 90.0 95.5 |
95.2 93.3 97.7 96.1 93.4 98.6 |
|
The back sections of the samples used in the ambient temperature
storage study were analyzed on days 10, 14, and 17 to determine if
migration from the sampling sections had occurred. No EGDN or NG was
found in the back sections.
4.8. Chromatograms
4.8.1. HPLC/UV Chromatogram
Figure 4.8.1. is a chromatogram obtained by the injection of 10
µL of a standard mixture containing the analytes. The HPLC column
was 4.6 mm × 25 cm DuPont Zorbax CN. The mobile phase was 90%
isooctane, 6% isopropanol, and 4% methanol. The flow rate was 1
mL/min. The UV detector wavelength was 214 nm.
4.8.2. HPLC/TEA Chromatogram
Figure 4.8.2. is a chromatogram obtained by the injection of 10
µL of a standard mixture containing the analytes. In this case, the
TEA was connected in series with a UV detector. The HPLC column and
mobile phase was the same as used in Figure 4.8.1.
4.9. Reproducibility study
Six vapor spiked large Tenax-GC tubes and a draft copy
of this evaluation were given to a chemist unassociated with this
work. The samples were analyzed after five days of storage at ambient
temperature. The results of the study are presented in Table 4.9.1.
The results are corrected for desorption efficiency.
Table 4.9.1. Reproducibility Study
|
amount vapor spiked, µg |
EGDN 18.6 |
NG 28.0 |
|
recovery, %
,
% SD, % |
103 103 100 103 98.3 112
103 4.7 |
97.5 93.9 85.4 100 89.6 101
94.6 6.1 |
|
4.10. Aerosol data
NG aerosols were generated by means of a Model 3050 Berland-Liu
Vibrating Orifice Monodisperse Aerosol Generator. The frequency of the
orifice was set at 7.5 KHz. An isopropanol solution containing 0.25
mg/mL of NG was metered into the system at 0.20 mL/min with a syringe
pump. The resulting stream was diluted with air (0.05
m3/min) to produce a 2.4-µm diameter
monodisperse aerosol containing 1 mg/m3 of
NG. The aerosol was sampled by means of ports connected to a sampling
chamber. Several experimental runs were performed to compare the
collection efficiencies of glass fiber filters, midget bubblers
containing ethanol, small Tenax-GC tubes and large
Tenax-GC tubes. The air sample volumes were about 15 L
and the sampling rate was 1 L/min.
NG aerosol samples were collected using small Tenax-GC
tubes to determine the location of the NG in the sampling tube. All
the NG was found on the front sorbent section. No NG was found on the
front glass wool plug, on the center polyurethane plug, on the
reference sorbent section, on the back polyurethane plug, or on the
glass tubing itself.
In another experiment, 3 glass fiber filters and 3 small
Tenax-GC resin tubes were used to sample the same NG
aerosol. The recovery of NG from the glass fiber filters was only 38%
of that recovered from the Tenax-GC tubes. A retention
efficiency experiment was later performed to determine if the filters
could retain NG. It was found that only 79% of the NG spiked on glass
fiber filters was retained after 14 L of air had been drawn through
the filter cassette. The aerosol and retention efficiency experiments
together indicate that the generated test aerosols probably have a
vapor component which was not collected on the filters.
The bubbler results were taken as the generation efficiency of the
aerosol apparatus because no NG was detected in backup bubblers or
backup Tenax-GC tubes. The generation efficiency was 75%.
The data in Table 4.10. are the results of 15 aerosol runs. Each
data point represents the average of at least two separate air samples
taken using identical sampling devices. The bubblers each contained 15
mL of ethanol as the trapping solution. Corrections for bubbler volume
and desorption efficiency were made in the reported results. The
results for the Tenax-GC tubes are expressed as ratios
relative to the bubbler results.
Table 4.10. Summary of NG Aerosol Results
|
run no. |
bubbler, mg/m3 |
(ratio) large
Tenax, mg/m3 bubbler,
mg/m3 |
(ratio) small
Tenax, mg/m3 bubbler,
mg/m3 |
|
1 2 3 4 5 6 7 8 9 10 11 12 13 14 15
SD CV |
0.77 0.77 0.78 0.78 0.81 0.78 0.79 0.76 0.80 0.78 0.74 0.68 0.81 0.56 0.69
0.75 0.066 0.087 |
---- ---- ---- ---- 0.79 ---- 0.99 0.95 0.82 0.90 0.99 0.97 0.93 0.95 0.92
0.92 0.068 0.074 |
0.91 1.1 0.96 0.73 ---- 1.0 1.0 0.95 0.92 0.97 1.1 1.2 1.1 0.99 1.0
1.0 0.11 0.11 |
| 4
The data in Table 4.10. indicate that the small
Tenax-GC tubes give slightly higher results than do the
large tubes. The significance of the difference between the ratio
means of the two adsorbent tube collection methods was tested using a
two-tailed Student-t distribution. This is a test of hypothesis
regarding the difference between the means of two normal populations
having unequal and unknown variances when the samples are small and of
different size. The critical region, at the 95% confidence level, was
determined to be T' greater than 2.07 and less than -2.07. The
calculated t' statistic was 2.12. These computations show that a small
but significant difference between the two collection methods exists.
The large tubes were selected in preference to the small tubes because
of their higher retention efficiency and lack of migration problems
for EGDN.
4.11. Vapor spiking technique
The Tenax-GC tubes were vapor spiked with EGDN and NG
by first injecting a liquid mixture of the analyte on a 1-cm plug of
silanized glass wool contained in an 8-mm i.d. × 40 mm long silane
treated glass tube. The spiked tube was placed in front of the
Tenax-GC tube and then air, at about 80% relative
humidity and 22°C, was drawn through the sampling train. The analytes
were vaporized from the glass wool and collected on the
Tenax-GC tube. The vaporization process was determined to
be complete after 25 L of air had passed through the sampling train.
Figure 4.1. Detection limits of the analytical
procedure. Peak identification was as follows: 1, EGDN; 2,
NG.
Figure 4.3.1. Calibration curve for
EGDN.
Figure 4.3.2. Calibration curve for NG.
Figure 4.7.1. Refrigerated temperature storage test for
EGDN.
Figure 4.7.2. Ambient temperature storage test for
EGDN.
Figure 4.7.3. Refrigerated temperature storage test for
NG.
Figure 4.7.4. Ambient temperature storage test for
NG.
Figure 4.8.1. HPLC/UV chromatogram. Peak identification was
as follows: 1, EGDN; 2, NG.
Figure 4.8.2. HPLC/TEA chromatogram. Peak identification was
as follows: 1, EGDN; 2, NG.
5. References
5.1. Hogstedt, C.; Davidsson, B. American Ind. Hygiene Assoc.
J. (1980), 41, 373.
5.2. Chrostowski, J.E.; Holmes, R.N.; Rehn, B.W. J. of Forensic
Sciences (1975), 611.
5.3. "NIOSH Manual of Analytical Methods", 2nd ed.; Department of
Health, Education and Welfare, National Institute for Occupational
Safety and Health; Cincinnati, OH. 1977; Vol. 3, Method No. S216; DHEW
(NIOSH) Publ. (U.S.), No. 77-1576.
5.4. "Profiling Common Explosives by LCEC" LCEC Applications Note
No. 32; Bioanalytical Systems Inc.; West Lafayette, IN.
5.5. McLinley, W.A. J. of Analytical Toxicology, (1981), 5,
209.
5.6. Krull, I.S.; Davis, E.A.; Santasania, C.; Krous, S.; Basch,
A.; Bemberger, Y. Analytical Letters (1981), 14, 1363.
5.7. Lafleur, A.L; Morriseau, B.D. Anal. Chem. (1980), 52,
1313.
5.8. "Documentation of the Threshold Limit Values", 4th ed.;
American Conference of Governmental Industrial Hygienists, Cincinnati,
OH, (1981), 184.
5.9. "General Industry" OSHA Safety and Health Standards (29 CFR
1910), U.S. Department of Labor, Occupational Safety and Health
Administration, OSHA 2206, Revised June, 1981.
5.10. "Criteria for a Recommended Standard....Occupational Exposure
to Nitroglycerin and Ethylene Glycol Dinitrate"; Department of Health,
Education and Welfare; National Institute for Occupational Safety and
Health; Cincinnati, OH. (1978); DHEW (NIOSH) Publ. (U.S.) No. 78-167.
5.11. Oketani, Y.; Mitsozono, T.; Ichikawa, K.; Itono, Y.; Gojo,
T.; Gofuku, M.; Konoha, N. Oyo Yakuri (1981), 22, 737.
5.12. Urbanski, T. "Chemistry and Technology of Explosives", Vol
II, Pergamon Press: Oxford, 1965, p. 36.
|