VOLATILE NITROSAMINE MIXTURE II |
|
N-nitrosomethylethylamine
(NMEA) N-nitrosodiisopropylamine
(NDiPA) N-Nitrosomethyl-n-butylamine
(NMBA) N-Nitrosoethyl-n-butylamine
(NEBA) N-Nitroso-n-propyl-n-butylamine
(NPBA) N-nitrosodiamylamine
(NDAmA) |
Method no.: |
38 |
|
Matrix: |
Air |
|
Procedure: |
Samples are collected using ThermoSorb/N air
samplers. The samples are desorbed with a dichloromethane/methanol
(75:25)(v/v) solution. Analysis is by gas chromatography and
detection with a Thermal Energy Analyzer. |
|
Recommended air volume and sampling rate: |
75 L at 1 L/min (0.2 to 2 L/min is
permissible) |
|
Special requirements: |
It is recommended that collected samples be stored in
a freezer. |
|
Status of method: |
A sampling and analytical method that has been
subjected to the established procedures of the Organic Methods
Evaluation Branch. |
|
Date: June 1982 |
Chemist: Warren
Hendricks |
Organic Methods Evaluation Branch OSHA Analytical
Laboratory Salt Lake City, Utah
Volatile Nitrosamine Mixture II
|
analyte |
NMEA |
NDiPA |
NMBA |
NEBA |
NPBA |
NDAmA |
|
target
concentration1 µg/m3 ppb
reliable
quantitation
limit µg/m3 ppb
standard
error of estimate at the target concentration, % (Section
4.7.) |
2.7 0.74
0.13 0.036
7.83 |
2.9 0.55
0.15 0.028
6.48 |
2.5 0.53
0.13 0.027
6.68 |
2.9 0.55
0.15 0.028
7.01 |
2.7 0.45
0.13 0.022
6.7 |
4.0 0.52
0.20 0.026
6.67 |
|
1 Air
concentrations are based on a 75-L air sample
volume. |
1. General Discussion
1.1. Background
1.1.1. History
The purpose of this work was to extend previously developed
volatile N-nitrosamine air sampling and analytical
techniques (Ref. 5.1.) to another set of N-nitrosamine
analytes. This air sampling procedure has now been validated for 13
N-nitrosamines.
Volatile N-nitrosamines have been collected using
cold traps (Ref. 5.2.), bubblers (Ref. 5.2.) and various solid
adsorbents (Ref. 5.4.). Most of these air sampling techniques have
serious deficiencies. The cold traps are difficult to maintain and
are reported to enable the artifactual (in situ) formation of the
analytes from precursor amines and nitrogen oxides (Ref. 5.5.).
Bubblers are inconvenient and analyte retention efficiency decreases
with increased sampling time and temperature (Ref. 5.4.). Solid
adsorbents, in general, may concentrate precursor amines and
nitrosating agents resulting in artifactual formation of the
analytes (Ref. 5.4.).
The commercially available ThermoSorb/N air sampling system is
reported to be free of the many problems associated with sampling
air for N-nitrosamines (Ref. 5.4.). The device is an
opaque plastic cartridge containing a solid adsorbent. The
manufacturer states that the device has adequate capacity to collect
most N-nitrosamines and also to prevent artifactual
formation of the analytes because of a proprietary amine trap and
nitrosation inhibitor (Ref. 5.4.). Since the device has been
successfully used by OSHA industrial hygienists to collect similar
analytes, it was decided to evaluate ThermoSorb/N cartridges as
sampling media for this method.
Previous analytical methods for N-nitrosamines
include polarographic (Ref. 5.6.), spectrophotometric (Ref. 5.6.),
and thin layer chromatographic (Ref. 5.6.) techniques. The analytes
have been determined both directly and following derivatization. The
main disadvantages of these methods are that they have limited
application to low level analysis of complex samples, they are only
semi-quantitative, they have insufficient resolution
and lack selective detection systems (Ref. 5.6).
Gas and liquid chromatographic analytical techniques have greater
application to trace level analysis of N-nitrosamines
than the methods previously mentioned because they provide better
analyte resolution and also because these methods utilize more
selective detectors (Ref. 5.6.).
The Thermal Energy Analyzer (TEA) is a highly selective detector
for N-nitroso compounds that has been successfully
interfaced to gas and liquid chromatographs. In the gas
chromatography (GC) mode of operation, the chromatographed
N-nitroso compound exits the GC and enters the TEA
pyrolyzer through a heated transfer line. The chemical bond between
the two nitrogen atoms (N-NO) is thermally broken, resulting in an
organic fragment and a nitrosyl radical (·NO). Organic
compounds, solvents and fragmentation products are collected in a
cold trap. The nitrosyl radical is a stable gas which passes through
the cold trap to react with ozone under vacuum to form
electronically excited nitrogen dioxide. The excited nitrogen
dioxide quickly decays to its ground state and emits light, at a
characteristic wavelength, which is measured by a photomultiplier
tube. When the TEA is used as a high performance liquid
chromatograph (HPLC) detector, the sequence of events is similar to
those of the GC mode. The chromatographed analyte exits the HPLC
column and enters the TEA pyrolyzer. A higher temperature pyrolyzer
is used because the HPLC mobile phase is flash vaporized at the same
time the N-nitroso bond is thermally broken. The
vaporized HPLC mobile phase, organic compounds and fragmentation
products are condensed in a cold trap. The nitrosyl radical is swept
through the cold trap with an inert gas, usually helium or argon,
and is detected as before (Ref. 5.7.).
This method uses a GC/TEA procedure for the analysis of the
component analytes. A HPLC/TEA procedure is also presented for use
as a confirmatory technique.
1.1.2. Toxic effects (This section is for information and should
not be taken as the basis of OSHA policy).
The effects of acute exposure to each of the analytes were
similar. The administration of a lethal dose to rats led to a
progressively weakened and emaciated condition frequently with the
appearance of jaundice. Death usually occurred within seven days.
The autopsy revealed severe centrilobular liver damage with
hemorrhaging into the lungs in most cases. The response to a lethal
dose was the same for male and female rats (Ref. 5.8.).
Each of the analytes evaluated in this method has a carcinogenic
effect on the rat. There is no evidence that exposure to the
analytes has led to cancer in humans and extrapolation of animal
data to humans is controversial, but experience with similar
N-nitroso compounds suggests that human exposure should
be kept to a minimum (Ref. 5.28.).
A high correlation between carcinogenicity and mutagenicity has
been reported (Ref. 5.10.). NDAmA, NMBA, and NPBA were found to be
mutagenic on Salmonella typhimurium when incubated with a rat
liver activating system (Ref. 5.11.). No information regarding the
mutagenicity of NMEA, NDiPA, and NEBA was obtained through a
literature search.
A generally accepted theory of N-nitrosamine
carcinogenesis proposes that the agents require biological
activation in order to exert their carcinogenic effects. If a
compound is directly active, local sarcomas should be observed at
the site of subcutaneous injection because of the high concentration
of the agent and sensitivity of the subcutaneous tissue. If,
however, local sarcomas do not develop and tumors in distant organs
are observed, the agent is an intermediate from which the actual
carcinogen is produced by metabolic processes. Subcutaneous
injection of N-nitrosodialkylamines did not result in
local sarcomas and the carcinogenic effects were evident in specific
organs (lungs, liver, bladder, etc.). The
N-nitrosodialkylamines are, therefore, not considered
to be the ultimate carcinogens (Ref. 5.8.).
The metabolic activation of a N-nitrosodialkylamine
initially involves the enzymatic hydroxylation of the carbon atom
immediately adjacent (in the alpha position) to the
N-nitroso group. This is followed by a series of other
steps, one of which is the formation of a diazoalkane which
ultimately leads to a carbonium ion (Figure 1.). A carbonium ion is
believed to be the carcinogenic species. This mechanism for the
formation of the carbonium ion is supported by the fact that
ethyl-tert-butylnitrosamine, which is structurally
unable to form the intermediate diazoalkane species, was found not
to cause cancer in rats, even at high doses. Exposure to a similar
compound, ethyl-n-butylnitrosamine, which structurally
can form a diazoalkane did lead to cancer in rats (Ref. 5.8.).
Detailed information regarding chronic exposure to the analytes
is presented in Section 4.9.
1.1.3. Potential workplace exposure
A literature search resulted in no citations regarding
occupational exposure to any of the analytes.
N-nitrosamines have been detected in various
industries (Refs. 5.12. - 5.14.) and industrial products (Refs.
5.12. and 5.15. - 5.22.).
Occupational exposure to the analytes is probably the result of
the formation of the agent from precursor amines and suitable
nitrosating species. The amino group can be primary, secondary or
tertiary (Ref. 5.25.). The amine can be free or a portion of a more
complex molecule such as a drug or a herbicide. Amines can be
nitrosated in air (Ref. 5.41.) or in solution under acidic, neutral
or alkaline conditions (Refs. 5.23. and 5.25.). The nitrosation
reaction is catalyzed by thiocyanate, halide ions, metal ions,
formaldehyde and ozone (Refs. 5.23., 5.25., and 5.31.). Suitable
nitrosating species include nitrogen oxides (NO,
NO2,
N2O4,
N2O3), nitrite
and nitrous acid (Refs. 5.23. and 5.25.).
Nitrosation can occur as a result of transnitrosation. This is a
chemical reaction in which a N-nitrosamine transfers
its nitroso group to another amine (Refs. 5.24. and 5.25.).
Exposure to the analytes includes the endogenous formation of the
agents in the human gastrointestinal tract. Precursor amines have
been shown to react with nitrite to form the corresponding
N-nitrosamines under conditions found in the mammalian
stomach (Ref. 5.30.). N-nitrosomorpholine has been
produced in vivo by mice gavaged with morpholine and later exposed
to nitrogen dioxide in inhalation chambers (Ref. 5.32.). The source
of volatile N-nitrosamines found in normal human feces,
urine and saliva has been suggested to be in vivo nitrosation of
ingested secondary and tertiary amines (Ref. 5.29 and 5.33. -
5.34.).
The size of the work population that is exposed is unknown. Since
amines and suitable nitrosating species are ubiquitous, the number
of potential exposures could be large (Refs. 5.3. and 5.25.).
1.1.4. Physical properties
The following data were taken from Refs. 5.8. and 5.26.
|
analyte |
NMEA |
NDiPA |
NEBA |
NDAmA |
|
CAS no. mol wt bp, °C (mm
Hg)
solubility in water, g/100 mL
UV absorption
data l max,
nm log e l max, nm log e |
10595-95-6 88.13 70
(35)
30
230 3.85 335 1.90 |
601-77-4 130.22 96 (28) 194.5
(760) 1.3
232 3.86 343 1.84 |
4549-44-4 130.22 94-96
(14)
1.2
231 3.88 337 1.94 |
13256-06-9 186.34 136
(14)
0.014
234 3.86 349 1.94 |
|
The following data were taken from Refs. 5.27. and 5.28.
|
analyte |
NMEA |
NDiPA |
NEBA |
NDAmA |
|
physical appearance density mp, °C |
yellow liquid 0.9448
(18/4)
|
yellow1 solid 0.9442
(20/4) 48 |
yellow1 liquid
|
yellow1 liquid
|
|
1 personal
observation |
The following data were taken from Refs. 5.26. and 5.37.
|
analyte |
NMBA |
NPBA |
|
CAS no. mol wt bp,
°C physical appearance |
7068-83-9 116.19 56 (1.5 mm
Hg) yellow1 liquid |
25413-64-3 144.25
yellow1 liquid |
|
1
personal observation |
synonyms (Ref. 5.26.)
N-nitrosomethylethylamine
ethylamine, N-methyl-N-nitroso;
ethylmethylnitrosamine; methylaethyl-nitrosamin
(German); N,N-methylethylnitrosamine;
N-methyl-N-nitrosoethylamine;
N-nitrosoethylmethylamine; NEMA; NMEA.
N-nitrosodiisopropylamine
diisopropylamine, N-nitroso; diisopropylnitrosamin
(German); NDiPA.
N-nitrosomethyl-n-butylamine
butylamine, N-methyl-N-nitroso-; butanamine,
N-methyl-N-nitroso-;
methyl-butyl-nitrosamin (German);
methylbutyl-nitrosamine;
methyl-n-butylnitrosamine;
N-methyl-N-nitrosobutylamine;
N-nitroso-n-butylmethylamine;
nitrosomethyl-n-butylamine; MBNA; NMBA.
N-nitrosoethyl-n-butylamine
aethyl-n-butyl-nitrosamin (German);
N-ethyl-N-nitrosobutylamine; butylamine,
N-ethyl-N-nitroso-; butanamine,
N-ethyl-N-nitroso-;
ethyl-n-butylnitrosamine; N-ethyl-N
nitrosobutylamine;
N-nitroso-n-butylethylamine; NEBA.
N-nitroso-n-propyl-n-butylamine
1-butanamine,N-nitroso-N-propyl-; butylamine,
N-nitroso-N-propyl-;
N-propyl-N-butylnitrosoamine; NPBA.
N-nitrosodiamylamine
dipentylamine, N-nitroso-; diamylnitrosamin
(German); di-n-amylnitrosamine;
di-n-pentylnitrosamine; NDAmA.
structure and molecular formula (Ref. 5.26.).
NMEA |
 |
C3H8N2O |
|
NDiPA |
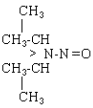 |
C6H14N2O |
|
NMBA |
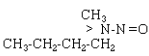 |
C5H12N2O |
|
NEBA |
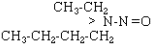 |
C6H14N2O |
|
NPBA |
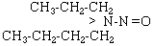 |
C7H16N2O |
|
NDAmA |
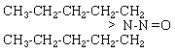 |
C10H22N2O |
1.2. Limit defining parameters (All air concentrations presented
are based on a 75 L air sample volume and a 1-mL
desorption volume.)
1.2.1. Detection limits of the analytical procedure
The detection limit of the analytical procedure is the mass of
analyte per injection which will result in a peak whose height is
about five times the amplitude of the baseline noise. (Section 4.1.)
Detection Limits of the Analytical Procedure
(pg/injection)
|
NMEA |
NDiPA |
NMBA |
NEBA |
NPBA |
NDAmA |
|
50 |
55 |
47 |
55 |
50 |
75 |
|
1.2.2. Detection limits of the overall procedure
The detection limit of the overall procedure is the amount of
analyte spiked on the sampling device which allows recovery of an
amount of analyte equivalent to the detection limit of the
analytical procedure. (Section 4.2.)
Detection Limits of the Overall Procedure
|
analyte |
NMEA |
NDiPA |
NMBA |
NEBA |
NPBA |
NDAmA |
|
ng/sample µg/m3 ppb |
10 0.13 0.036 |
11 0.15 0.028 |
9.4 0.13 0.027 |
11 0.15 0.028 |
10 0.13 0.022 |
15 0.20 0.026 |
|
1.2.3. Reliable quantitation limits
The reliable quantitation limit is the smallest amount of analyte
which can be quantitated within the requirements of at least 75%
recovery and a precision (±1.96 SD) of ±25% or better, based on six
samples.
The reliable quantitation limits were the same as the detection
limits of the overall procedure since the desorption efficiencies
were above 75% and the precisions were better than ±25%. (Section
4.2.)
Reliable Quantitation Limits
|
analyte |
NMEA |
NDiPA |
NMBA |
NEBA |
NPBA |
NDAmA |
|
ng/sample µg/m3 ppb |
10 0.13 0.036 |
11 0.15 0.028 |
9.4 0.13 0.027 |
11 0.15 0.028 |
10 0.13 0.022 |
15 0.20 0.026 |
|
The reliable quantitation limit and detection limits reported in
the method are based upon optimization of the instrument for the
smallest possible amount of analyte. When the target concentration of
an analyte is exceptionally higher than these limits, they may not be
attainable at the routine operating parameters.
1.2.4. Sensitivity
The sensitivity of the analytical procedure is determined by the
slope of the calibration curve over a concentration range from 0.5
to 2 times the target concentration. The sensitivity will vary
somewhat with the particular instrument used in the analysis.
(Section 4.4.)
Sensitivity of the Analytical Procedure (area units per
µg/mL)
|
NMEA |
NDiPA |
NMBA |
NEBA |
NPBA |
NDAmA |
|
279441 |
204894 |
192333 |
231792 |
174773 |
114093 |
|
1.2.5. Recovery
The recovery of analyte from the collection medium during storage
must be 75% or greater. The minimum recoveries which are presented
below were determined from the regression line of the plotted
ambient temperature storage data. (Section 4.7.)
Recovery (%)
|
NMEA |
NDiPA |
NMBA |
NEBA |
NPBA |
NDAmA |
|
93.6 |
97.6 |
93.6 |
93.3 |
90.6 |
93.4 |
|
1.2.6. Precision (analytical method only)
The pooled coefficients of variation obtained from replicate
determinations of analytical standards at 0.5, 1 and 2 times the
target concentrations are presented below. (Section 4.3.)
The Pooled Coefficients of Variation
|
NMEA |
NDiPA |
NMBA |
NEBA |
NPBA |
NDAmA |
|
0.013 |
0.018 |
0.020 |
0.026 |
0.017 |
0.025 |
|
1.2.7. Precision (overall procedure)
The overall procedure must provide results at the target
concentrations that are ±25% or better at the 95% confidence level.
The precisions at the 95% confidence level for the
16-day storage tests are presented below (Section 4.7.,
Figures 4.7.1. - 4.7.6.). The reported values each include an
additional ±5% for sampling error.
Precision (%) of the Overall Procedure
|
NMEA |
NDiPA |
NMBA |
NEBA |
NPBA |
NDAmA |
|
15.3 |
12.7 |
13.0 |
13.7 |
13.1 |
13.0 |
|
1.2.8. Reproducibility
Six vapor-spiked samples and a draft copy of this
procedure were given to a chemist unassociated with this evaluation.
The samples were analyzed after one day of storage at ambient
temperature. The average recoveries and standard deviations are
presented below (Section 4.10.). The samples were spiked at the
target concentrations.
Reproducibility Study
|
analyte |
NMEA |
NDiPA |
NMBA |
NEBA |
NPBA |
NDAmA |
|
amount vapor spiked,
µg
, % SD, % |
0.20
100.8 6.65 |
0.22
99.2 5.29 |
0.19
100.9 3.99 |
0.22
100.0 2.85 |
0.20
103.4 4.01 |
0.30
104.4 3.45 |
|
1.3. Advantages
1.3.1. The sampling and analytical procedures permit the
simultaneous determination of a mixture of analytes.
1.3.2. The sampling and analytical procedures are precise,
reliable, safe and convenient.
1.3.3. The sampling procedure is artifact free under the tested
conditions. The capacity of the sampling device to prevent artifacts
is probably limited.
1.3.4. The air sampling device is commercially available and is
constructed of opaque plastic to prevent
photo-degradation of the collected
N-nitrosamines.
1.3.5. The samples are stable when stored at ambient temperatures
for at least 16 days.
1.4. Disadvantages
1.4.1. Smaller laboratories may not be able to support the cost
of the recommended sampling and analytical instruments.
1.4.2. The ability of the sampling device to collect and retain
the analytes is limited.
2. Sampling Procedure
2.1. Apparatus
2.1.1. An air sampling pump, the flow of which can be determined
to within ±5% at the recommended air flow rate with the air sampler
in line.
2.1.2. ThermoSorb/N air sampling cartridges, available from
Thermo Electron Corporation, Waltham, Mass.
2.1.3. Equipment to calibrate the air flow rate through the
sampling device.
2.2. Reagents
None required
2.3. Technique
2.3.1. Prior to sampling, remove the ThermoSorb/N air sampling
device from the foil container. Save the container for reuse.
2.3.2. Prior to sampling, remove the red end caps from the inlet
and outlet ports. Store the caps on the air sampler in the places
that are provided for this use.
2.3.3. Label the air sampler and attach the device to the air
sampling pump with flexible tubing. Adjust the pump to obtain the
proper air flow rate. The recommended rate is 1 L/min but flow rates
of from 0.2 to 2 L/min may be used. If air volumes larger than the
recommended 75 L are required or if large amounts of
N-nitrosamines are suspected, two air samplers may be
connected in series. Always calibrate the pump with the sampling
device in line.
2.3.4. Attach the sampling device in the breathing zone of the
employee to be monitored. The molded clip is convenient for this
purpose.
2.3.5. After sampling for the appropriate time, remove the device
and replace the red end caps on the inlet and outlet ports of
sampler.
2.3.6. Wrap each sample end to end with official OSHA seals (Form
21). Place the sealed air sampler inside the foil container from
Section 2.3.1.
2.3.7. With each set of samples, submit at least one blank
sample. The blank should be subjected to the same handling as the
sample except that no air is drawn through it.
2.3.8. Place the samples in a freezer if they are to be stored
before shipping to the laboratory.
2.3.9. List possible interferences on the sample data sheet.
2.4. Breakthrough
Breakthrough studies were conducted by connecting two samplers in
series. The first sampler was vapor spiked at twice the target
concentrations with a mixture of the analytes and then air at about
80% relative humidity and 22°C was drawn through the sampling train.
The rear sampler was removed and analyzed after an appropriate air
volume had been sampled. The rear sampler was replaced with a new
cartridge and sampling was continued. The first tube was also analyzed
when the study was terminated.
NMEA was the only component of the mixture to be lost from the
first sampling device. Two breakthrough studies were conducted and the
average 5% breakthrough air volume was 169 L (Section 4.5.). The 5%
breakthrough air volume was defined as the air volume at which 5% of
the amount of the component vapor spiked on the first tube was found
on the second tube. None of the other analytes moved from the front
tube after 365 L of air was drawn through the sampling train.
In the interests of a general N-nitrosamine air
sampling procedure, 75 L was selected as the recommended air volume
for this method because previously evaluated
N-nitrosamines have been shown to have a lower 5%
breakthrough air volume than NMEA. (Ref. 5.1.)
2.5. Desorption efficiency
The average desorption efficiency for each of the analytes vapor
spiked at 0.5, 1 and 2 times the target concentration on ThermoSorb/N
air samplers is presented below (Section 4.6.).
Average Desorption Efficiencies, %
|
NMEA |
NDiPA |
NMBA |
NEBA |
NPBA |
NDAmA |
|
101.1 |
100.7 |
100.7 |
99.5 |
99.5 |
95.7 |
|
2.6. Recommended air volume and sampling rate
2.6.1. The recommended air volume is 75 L.
2.6.2. The recommended air sampling rate is 1 L/min. Studies
indicate that flow rates of from 0.2 to 2 L/min may be used if
required.
2.7. Interferences (sampling)
2.7.1. The commercial ThermoSorb/N N-nitrosamine
air sampler is constructed to prevent the in situ formation of
N-nitrosamines from airborne precursors. The
manufacturer has tested the sampling device for the artifactual
formation of N-nitrosodimethylamine (NDMA),
N-nitrosopiperidine (NPIP),
N-nitrosopyrrolidine (NPYR),
N-nitrosomorpholine (NMOR) and NDiPA using 50 µg each
of the respective precursor secondary amines and nitrogen oxides
(NOx). The amines were
pre-loaded on the ThermoSorb/N tubes and then 100 L of
air containing 0.2, 0.5, 1, 2 or 4 ppm
NO/NO2 at a 1/1 ratio were sampled.
Various untreated solid adsorbents formed the
N-nitrosamines quite easily but at these levels of
amines and NOx, the ThermoSorb/N
cartridges were shown to be artifact free (Ref. 5.4.).
The process by which the ThermoSorb/N tubes prevent artifact
formation is proprietary. The manufacturer states that the device
has an amine trap that removes incoming amines and holds them
unavailable for nitrosation. The sampled air, after passing through
the amine trap, enters a solid sorbent bed where the
N-nitrosamines are quantitatively removed and retained.
The sampled air then passes through fiber pads containing a
nitrosation inhibitor and finally exits the sampler. When the air
sampling device is backflushed with solvent, the nitrosation
inhibitor is put into solution and is washed together with the
collected N-nitrosamines and amines into a collection
vial. The nitrosation inhibitor successfully competes with the amine
and chemically removes any nitrosating species (Ref. 5.35.).
The resistance of the ThermoSorb/N air sampler to artifact
formation was verified using morpholine (a precursor amine for NMOR)
and nitrogen dioxide. Morpholine was selected as the test amine
because it has been shown to be easily nitrosated. Nitrogen dioxide
in a gas bag, mixed with humid air, has been shown to be an
effective nitrosating species. A Teflon gas bag was prepared
containing 33 µg/L morpholine in nitrogen and another containing 4.8
ppm v/v NO2 in air at about 75% relative
humidity. The morpholine bag was sampled for 50 min at 1 L/min and
then the nitrogen dioxide bag was sampled for 50 min at 1 L/min. The
total morpholine loaded on the tube was 1650 µg. There was no NMOR
formed on the ThermoSorb/N cartridge. The experiment was repeated
using a standard Florisil tube. The result showed that 7 µg of NMOR
could be formed, as an artifact on the tube, after sampling each bag
for 7 min.
2.7.2. It is unknown if there are other interferences with the
collection of N-nitrosamines using ThermoSorb/N
cartridges.
2.8. Safety precautions (sampling)
2.8.1. Attach the sampling equipment to the worker so it will
not interfere with work performance or safety.
2.8.2. Follow all safety practices that apply to the work area to
be monitored.
3. Analytical Procedure
3.1. Apparatus
3.1.1. A temperature programmable gas chromatograph (GC).
3.1.2. A high performance liquid chromatographic (HPLC) pump.
3.1.3. A HPLC sample injector.
3.1.4. A Thermal Energy Analyzer (TEA), Thermo Electron
Corporation, Waltham, Mass.
3.1.5. A GC column capable of resolving the analytes from each
other and potential interferences. The column used in this work was
10-ft × 1/8-in. stainless steel containing 10% Carbowax
20M with 2% KOH on 80/100 mesh Chromosorb W AW.
3.1.6. A HPLC analytical column capable of resolving the analytes
from each other and potential interferences. The column used in this
work was a DuPont Zorbax CN (4.6 mm × 25-cm).
3.1.7. The necessary hardware to interface the TEA to the GC and
the HPLC apparatus.
3.1.8. An electronic integrator or other suitable means to
measure peak area and record chromatograms. A
Hewlett-Packard 3354 B/C data system was used for this
evaluation. The A/D converter was set for 2 readings per second.
3.1.9. Vials, 2-mL with Teflon-lined
caps.
3.1.10. Syringes, of convenient sizes for sample and standard
preparations and injections.
3.1.11. Hypodermic needles, 23 gauge × 1 in., Luer hub.
3.1.12. Volumetric flasks, 1-mL and other convenient
sizes.
3.1.13. Dewar flasks, of convenient sizes for liquid nitrogen.
3.1.14. Microanalytical balance.
3.2. Reagents
3.2.1. Analytical standards: NMEA, NDiPA, NMBA, NEBA, NPBA and
NDAmA.
3.2.2. Dichloromethane and methanol, HPLC grade. The sample
desorption solution is composed of 75% dichloromethane and 25%
methanol by volume.
3.2.3. Isopropanol and 2,2,4-trimethylpentane, HPLC
grade.
3.2.4. n-Propanol, technical grade.
3.2.5. Nitrogen, liquid.
3.2.6. Helium, GC grade.
3.2.7. Oxygen and air, medical grade.
3.3. Standard preparation
3.3.1. Keep the exposure of the standards to light at a minimum
because light will decompose each of the analytes.
3.3.2. Prepare individual stock standards of by diluting known
quantities of each component with isopropanol.
3.3.3. Prepare an intermediate standard mixture using known
volumes of each stock standard and diluting the mixture with
2,2,4-trimethylpentane. The following intermediate
standard mixture was used for this evaluation.
Concentration of the Standard Mixture, µg/mL
|
NMEA |
NDiPA |
NMBA |
NEBA |
NPBA |
NDAmA |
|
10 |
11 |
9.4 |
11 |
10 |
15 |
|
3.3.4. Prepare fresh working range standards daily by diluting
the standard mixture with desorbing solution. Standards at the
target concentration were obtained by diluting the above standard
mixture 1 to 50 with desorbing solution.
3.3.5. Additional standards at other than the target
concentration should be prepared in order to generate the
calibration curve.
3.3.6. Store the standards in a freezer using
well-sealed, dark containers.
3.4. Sample preparation
3.4.1. Store the samples in a freezer until analysis.
3.4.2. The sample should be received in a foil container. Remove
the sample from the container.
3.4.3. Insure that the official OSHA seal (Form 21) is intact and
complete.
3.4.4. Check the laboratory sample number against the field
identification number to be sure that the sample has been properly
identified.
3.4.5. Prepare the desorption solution described in Section
3.2.2. Store the solution in a well sealed, dark bottle.
3.4.6. Label two 1-mL volumetric flasks with the
sample number. Further label one flask "A" and the other "B".
3.4.7. Remove the OSHA seal and the red end caps from the sample.
3.4.8. Attach a syringe needle to the male Luer fitting at the
inlet port of the air sampler.
3.4.9. Fill a syringe with about 4 mL of the desorbing solution.
Attach the syringe to the female Luer fitting located at the outlet
end of the air sampler.
3.4.10. Elute the sample by gently forcing the desorption solvent
through the air sampler at approximately 0.5 mL/min. Collect the
first 1-mL portion of solvent in the volumetric flask
labeled "A" in Section 3.4.6. and the second 1 mL of eluant in the
flask labeled "B".
3.4.11. Because light will decompose N-nitrosamines,
it is necessary to prevent exposure of the eluted samples to light.
3.4.12. If the eluted samples are not to be analyzed immediately,
transfer the contents of each flask to a separate vial which can be
sealed with a Teflon-lined cap. Protect the samples
from light and store them in a freezer.
3.5. Analysis
3.5.1. Instrument conditions
3.5.1.1. GC conditions
injector temperature: |
150°C |
column temperature: |
150 to 220°C at 4°C/min |
carrier gas flow rate: |
30 mL/min (helium) |
injection volume: |
5 µL |
The recommended GC column is 10-ft × 1/8-in.
stainless steel packed with 10% Carbowax 20M with 2% KOH on 80/100
mesh Chromosorb W AW.
3.5.1.2. TEA Conditions
The following conditions apply to the Model
TEA-502 with the retrofit Explosives Analysis Package
(EAP).
GC transfer temperature: |
220°C |
GC pyrolyzer temperature: |
500°C |
oxygen: |
5 mL/min |
attenuation: |
4 |
cold trap temperature: |
-130°C |
(n-propanol and liquid
nitrogen) |
3.5.2. Chromatogram Section 4.8.
3.5.3. Detector response is measured with an electronic
integrator or other suitable means.
3.5.4. An external standard procedure is used to prepare a
calibration curve using at least 3 standard solutions of different
concentrations. The calibration curve is prepared daily. The
integrator is calibrated to report results in µg/mL.
3.5.5. Bracket the samples with analytical standards.
3.6. Interferences (analytical)
3.6.1. N-nitrosodipropylamine has the same general
GC retention time as NMBA (Figure 4.8.2.). These analytes may be
resolved on a 1/8-in. × 10-ft stainless steel GC column
packed with 20% Versamid 900 on 80/100 Chromosorb W AW (Figure
4.8.3.). If either of these analytes are detected in samples, the
identity of the peak in question must be confirmed.
3.6.2. Because the TEA has been shown to respond to compounds
other than N-nitrosamines, it is strongly recommended
that positive GC/TEA results be confirmed by HPLC/TEA analysis.
Since GC and HPLC separation techniques operate using different
principles, the component elution order is not the same. It is
unlikely that two different compounds will have a coincidence of
retention time on both GC and HPLC columns.
3.6.2.1. HPLC conditions
column: |
DuPont Zorbax CN (4.6 mm × 25 cm) |
mobile phase: |
94.5% 2,2,4-trimethylpentane/5%
dichloromethane/0.5% isopropanol (v/v/v) |
flow rate: |
1.3 mL/min |
injection volume: |
5 to 25 µL |
The TEA conditions are the same as for the GC/TEA analysis
except that the HPLC pyrolyzer is used at 550°C and the cold trap
is maintained at -80°C with a mixture of water,
n-propanol and liquid nitrogen.
3.6.2.2. Chromatogram Figure 4.8.4.
3.6.3. GC and HPLC parameters may be changed to circumvent
interferences. Possible interferences are listed on the sample data
sheets.
3.6.4. The only unequivocal means of structure designation is by
gas chromatography/mass spectrometry with continuous peak matching.
It is recommended this procedure be used to confirm samples whenever
possible.
3.7. Calculations
3.7.1. The integrator value in µg/mL is used for reference only.
More reliable results are obtained by use of a calibration curve.
The detector response, for each standard, is compared to its
equivalent concentration in µg/mL and the best straight line through
the data points is determined by linear regression.
3.7.2. The concentration, in µg/mL, for a particular
determination is obtained by comparing its detector response to the
calibration curve.
3.7.3. The result obtained from the analysis of each vial or
flask is corrected by the appropriate desorption efficiency, and
then the corrected results from the "A" and "B" determinations that
compose a particular air sample are added together.
3.7.4. The analyte air concentrations for a sample are calculated
by the following equation:
concentration in µg/m3 = (C)(D)(1000)/E
where |
C |
= |
µg/mL from Section 3.7.3. |
|
D |
= |
desorption volume in milliliters (1 mL) |
|
E |
= |
air volume in liters |
3.7.5. To convert the results from Section 3.7.4. to parts per
billion (at 760 mm and 25°C) the following relationship is used:
concentration in ppb =
(µg/m3)(24.46)/MW
where |
µg/m3 |
= |
result from Section 3.7.4. |
|
24.46 |
= |
molar volume of an ideal gas at 25°C and 760
mm Hg. |
|
MW |
= |
molecular weight of the analyte, obtained
below. |
Molecular Weights
|
NMEA |
NDiPA |
NMBA |
NEBA |
NPBA |
NDAmA |
|
88.13 |
130.22 |
116.19 |
130.22 |
144.25 |
186.34 |
|
3.8. Safety precautions (analytical)
3.8.1. The analytes are extremely potent animal carcinogens and
utmost care must be exercised when working with these compounds.
3.8.2. Avoid skin contact with liquid nitrogen and the solvents.
3.8.3. Confine the use of solvents to a fume hood.
3.8.4. Wear safety glasses in all laboratory areas.
3.8.5. Check to be sure that the TEA exhaust is connected to a
fume hood.
4. Backup Data
4.1. Detection limit of the analytical procedure
Figure 4.1. is a chromatogram obtained from a 5-µL
injection of a standard solution at the following concentrations:
Concentrations of the Detection Limit Standard in
µg/mL
|
NMEA |
NDiPA |
NMBA |
NEBA |
NPBA |
NDAmA |
|
0.010 |
0.011 |
0.0094 |
0.011 |
0.010 |
0.015 |
|
Procedure used to Vapor Spike ThermoSorb/N Tubes
Each ThermoSorb/N tube was vapor spiked with the analytes by first
injecting a liquid mixture of the analytes on Polar Partition resin.
The spiked resin tube was then placed in front of the ThermoSorb/N
cartridge and 50 L (1 L/min) of air, at about 80% relative humidity
and 22°C, were drawn through the sampling train. The analytes were
vaporized from the resin and collected on the ThermoSorb/N tube. The
vaporization process was determined to be complete after 25 L of air
had passed through the resin.
4.2. The detection limit of the overall procedure and the reliable
quantitation limit
The following data were obtained by vapor spiking the analytes on
air samplers. The injection size recommended in the analytical
procedure (5-µL) was used to determine the detection limit of the
overall procedure and the reliable quantitation limit.
Table 4.2. Desorption Efficiency at the Detection
Limit
|
analyte ng/sample |
NMEA 10 |
NDiPA 11 |
NMBA 9.4 |
NEBA 11 |
NPBA 10 |
NDAmA 15 |
|
recovery, %
 SD 1.96
SD |
95.6 92.7 102.0 91.5 86.9 99.1
94.6 5.45 10.7 |
94.8 88.2 85.0 103.9 97.2 97.5
94.4 6.85 13.4 |
95.2 87.3 83.9 100.0 95.4 85.1
91.2 6.58 12.9 |
94.9 99.9 95.2 98.8 102.1 105.9
99.5 4.20 8.22 |
83.2 95.1 104.5 94.4 102.7 102.6
97.1 8.01 15.7 |
107.2 107.0 98.5 105.1 101.9 100.6
103.4 3.59 7.03 |
|
Since the desorption efficiencies were near 100% and also the
precisions were better than ±25%, the detection limits of the overall
procedure and the reliable quantitation limits were the same.
4.3. Precision data
The following data were obtained from multiple injections of
analytical standards:
Table 4.3.1. Precision at 0.5× Target
Concentration
|
analyte µg/mL |
NMEA 0.10 |
NDiPA 0.11 |
NMBA 0.094 |
NEBA 0.11 |
NPBA 0.10 |
NDAmA 0.15 |
|
area
counts
 SD CV |
27065 27193 27690 27758 27851
27511.4 356.612 0.0130 |
22586 22420 22124 22999 22323
22490.4 329.788 0.0147 |
18524 19648 19363 19412 19727
19334.8 478.557 0.0248 |
25698 23684 25931 25189 24922
25084.8 879.157 0.0350 |
18686 17699 18217 18418 18052
18214.4 372.786 0.0205 |
19604 19083 18838 18479 19805
19161.8 544.637 0.0284 |
|
Table 4.3.2. Precision at 1× Target Concentration
|
analyte µg/mL |
NMEA 0.20 |
NDiPA 0.22 |
NMBA 0.19 |
NEBA 0.22 |
NPBA 0.20 |
NDAmA 0.30 |
|
area
counts
 SD CV |
52963 53081 54020 54539 53835
53687.6 661.458 0.0123 |
44183 44391 43683 42981 42851
43617.8 691.924 0.0159 |
38450 37254 36628 38216 37771
37663.8 737.453 0.0196 |
47220 49112 47969 48028 46244
47714.6 1063.36 0.0223 |
34422 34512 33540 34727 34285
34297.2 452.753 0.0132 |
35067 34259 35449 35120 36502
35279.4 811.673 0.0230 |
|
Table 4.3.3. Precision at 2× Target Concentration
|
analyte µg/mL |
NMEA 0.40 |
NDiPA 0.44 |
NMBA 0.38 |
NEBA 0.44 |
NPBA 0.40 |
NDAmA 0.60 |
|
area
counts
 SD CV |
111830 108836 110374 112323 111588
110990.2 1401.72 0.0126 |
87667 89592 87938 92364 91555
89823.2 2104.25 0.0234 |
73717 75536 73669 73607 75048
74315.4 908.889 0.0122 |
1011134 100335 98393 102487 102664
101002.6 1749.05 0.0173 |
70263 69945 68823 71697 71112
70368.0 1106.43 0.0157 |
69269 69635 72803 71145 68670
70304.4 1668.91 0.0237 |
|
Table 4.3.4. The Pooled Coefficients of Variation
|
NMEA |
NDiPA |
NMBA |
NEBA |
NPBA |
NDAmA |
|
0.013 |
0.018 |
0.020 |
0.026 |
0.017 |
0.025 |
|
4.4. Sensitivity
The data in Tables 4.3.1. - 4.3.3. are presented graphically in
Figures 4.4.1. - 4.4.6. The sensitivity of the analytical procedure is
determined by the slope of the calibration curve over a concentration
range of from 0.5 to 2 times the target concentrations.
4.5. Breakthrough
Two breakthrough studies were conducted at about 80% relative
humidity and 22°C. Two ThermoSorb/N tubes were connected in series and
the front tube was vapor spiked at 2 times the target concentration
with a mixture of the analytes. The rear tube was removed and replaced
at intervals and analyzed. NMEA was the only analyte to move from the
front to the rear tube. The data is presented in Tables 4.5.1. and
4.5.2. and also in Figures 4.5.1. and 4.5.2.
Table 4.5.1. Breakthrough Study One
|
air sampled L |
NMEA recovery, % |
cumulative
NMEA recovery, % |
|
100 150 200 250 300 front tube |
ND 2.8 24.3 36.4 26.0 10.5 |
0.0 2.8 27.1 63.5 89.5 |
|
Table 4.5.2. Breakthrough Study Two
|
air sampled L |
NMEA recovery, % |
cumulative
NMEA recovery, % |
|
123 174 229 275 365 front tube |
ND 2.0 19.1 35.5 43.4 ND |
0.0 2.0 21.1 56.6 100.0 |
|
4.6. Desorption efficiency
The following data represent the analysis of ThermoSorb/N tubes
vapor spiked with the analytes at 0.5, 1, and 2 times the target
concentrations.
Table 4.6.1. Desorption Efficiency at 0.5× the Target
Concentration
|
analyte ng/sample |
NMEA 100 |
NDiPA 110 |
NMBA 94 |
NEBA 110 |
NPBA 100 |
NDAmA 150 |
|
% recovery
 |
98.4 104.2 97.9 103.6 105.2 98.6
101.3 |
94.1 93.9 102.8 102.2 111.0 94.2
99.7 |
99.0 88.6 103.2 96.6 112.1 97.1
99.4 |
99.4 89.2 97.9 91.6 105.5 96.2
96.6 |
92.8 84.5 98.5 97.9 109.1 100.5
97.2 |
102.3 99.6 104.2 91.0 94.7 101.0
98.8 |
|
Table 4.6.2. Desorption Efficiency at 1× the Target
Concentration
|
analyte ng/sample |
NMEA 200 |
NDiPA 220 |
NMBA 188 |
NEBA 220 |
NPBA 200 |
NDAmA 300 |
|
% recovery
 |
92.0 105.3 105.1 100.0 98.7 106.7
101.3 |
92.4 105.4 106.8 100.6 97.5 104.0
101.1 |
90.8 106.2 106.0 104.4 95.4 104.5
101.2 |
92.9 106.9 105.7 102.1 94.4 104.5
101.1 |
96.6 104.0 105.1 100.0 95.4 101.8
100.5 |
95.4 96.3 93.7 90.2 99.6 96.0
95.2 |
|
Table 4.6.3. Desorption Efficiency at 2× the Target
Concentration
|
analyte ng/sample |
NMEA 400 |
NDiPA 440 |
NMBA 376 |
NEBA 440 |
NPBA 400 |
NDAmA 600 |
|
% recovery
 |
101.3 102.1 103.2 103.8 98.0 96.5
100.8 |
101.5 103.7 100.8 100.0 104.9 97.8
101.4 |
102.6 102.6 99.8 101.4 103.9 99.3
101.6 |
101.6 103.0 97.8 101.2 102.4 99.5
100.9 |
100.9 102.2 100.1 102.0 104.4 95.2
100.8 |
87.0 92.5 93.6 95.5 94.6 94.8
93.0 |
|
Table 4.6.4. Average Desorption Efficiency at 0.5, 1,
and 2 Times the Target Concentration
|
NMEA |
NDiPA |
NMBA |
NEBA |
NPBA |
NDAmA |
|
101.1 |
100.7 |
100.7 |
99.5 |
99.5 |
95.7 |
|
4.7. Storage data
The data in Tables 4.7.1. and 4.7.2. represent the effects of
storage at ambient (21 to 26°C) and reduced (-20°C) temperatures on
vapor spiked ThermoSorb/N cartridges. The tubes were vapor spiked at
the following levels. The results are not corrected for desorption
efficiency. The data are also presented graphically in Figures 4.7.1.
to 4.7.12.
Amount Vapor spiked, ug/Cartridge
|
NMEA |
NDiPA |
NMBA |
NEBA |
NPBA |
NDAmA |
|
0.20 |
0.22 |
0.19 |
0.22 |
0.20 |
0.30 |
|
Table 4.7.1. Ambient Temperature Storage, %
Recovery
|
day |
sample |
NMEA |
NDiPA |
NMBA |
NEBA |
NPBA |
NDAmA |
|
0
3
6
9
13
16 |
1 2 3
1 2 3
1 2 3
1 2 3
1 2 3
1 2 3 |
105.8 102.8 105.1
96.1 99.9 104.2
85.9 86.4 92.8
91.8 96.9 91.0
103.8 95.4 92.0
95.5 100.6 93.2 |
105.4 101.5 104.8
102.8 108.5 104.7
94.0 90.2 98.1
100.2 101.9 98.5
101.9 98.3 100.9
98.1 100.8 95.1 |
105.8 100.2 104.0
98.2 105.0 97.1
92.4 87.5 95.9
94.8 95.2 92.9
100.8 98.4 96.0
95.0 97.0 90.2 |
104.2 98.6 104.5
100.4 107.2 104.0
89.7 86.2 95.4
99.3 98.8 98.2
94.2 92.2 97.1
93.4 99.0 91.8 |
104.2 101.0 105.1
96.1 105.0 102.0
89.8 88.2 98.1
97.1 96.9 95.0
91.6 88.4 89.5
93.6 98.5 89.6 |
92.0 97.3 98.4
91.3 102.7 99.4
88.8 85.4 94.4
90.7 93.4 89.7
93.0 93.3 94.3
94.0 100.0 94.4 |
|
Table 4.7.2. Reduced Temperature Storage, %
Recovery
|
day |
sample |
NMEA |
NDiPA |
NMBA |
NEBA |
NPBA |
NDAmA |
|
0
3
6
9
13
16 |
1 2 3
1 2 3
1 2 3
1 2 3
1 2 3
1 2 3 |
99.0 94.4 91.6
98.0 93.8 99.3
94.2 100.2 97.3
100.6 101.6 100.6
103.1 92.8 97.0
91.1 92.1 93.2 |
100.8 94.9 101.9
100.4 99.8 99.7
100.4 101.7 99.9
103.9 99.5 101.1
104.8 95.2 98.8
95.1 99.1 97.4 |
102.6 94.3 98.4
97.6 98.0 97.7
98.7 97.6 97.1
99.1 97.2 95.8
98.6 91.9 89.8
90.2 94.2 96.9 |
103.5 94.5 98.5
99.5 100.0 98.3
99.3 100.1 98.2
104.4 99.0 97.4
98.7 94.8 94.1
88.8 92.6 94.2 |
103.3 93.6 95.0
103.5 101.5 97.0
97.5 93.6 89.6
101.2 91.4 92.7
101.1 94.5 99.4
91.0 94.2 93.3 |
101.1 91.3 86.8
87.7 98.1 97.8
94.4 96.8 98.3
88.4 91.4 95.1
96.4 88.2 103.4
93.4 93.4 93.2 |
|
4.8. Chromatograms
4.8.1. GC/TEA Chromatogram
Figure 4.8.1. is a typical GC/TEA chromatogram obtained by the
injection of a standard mixture containing the analytes. The column
was 1/8-in. × 10-ft stainless steel packed with 10%
Carbowax 20M with 2% KOH on 80/100 Chromosorb W AW.
4.8.2. GC/TEA Chromatogram
Figure 4.8.2. is a GC/TEA chromatogram obtained from the analysis
of a mixture of N-nitrosamines. The analytes are those
of Volatile Mixtures I and II. Note that NMBA and
N-nitrosodipropylamine are not resolved. The analytical
column was 1/8-in. × 10-ft stainless steel packed with
10% Carbowax 20M with 2% KOH on 80/100 Chromosorb W AW.
N-nitrosamines of Volatile Mixture I shown in
Figures 4.8.2.-4.8.4. are: N-nitrosodimethylamine
(NDMA); N-nitrosodiethylamine (NDEA);
N-nitrosodipropylamine (NDPA);
N-nitrosodibutylamine (NDBA);
N-nitrosopiperidine (NPIP);
N-nitrosopyrrolidine (NPYR);
N-nitrosomorpholine (NMOR).
4.8.3. GC/TEA Chromatogram
Figure 4.8.3. is a GC/TEA chromatogram obtained by the analysis
of the same N-nitrosamine mixture as used in Section
4.8.2. on a different GC column. Note that while NMBA and NDPA are
resolved, NPIP and NPYR are not resolved. The column was 1/8-in. ×
10-ft stainless steel packed with 20% Versamid 900 on
80/100 Chromosorb W AW.
4.8.4. HPLC/TEA Chromatogram
Figure 4.8.4. is a HPLC/TEA chromatogram obtained by the
injection of the same standard mixture used in 4.8.2. The column was
4.6-mm i.d. × 25-cm DuPont Zorbax CN. The mobile phase
was 94.5% 2,2,4-trimethylpentane, 5% dichloromethane
and 0.5% isopropanol. The flow rate was 1.3 mL/min.
4.9. Toxic effects (This data is for information only and should
not be taken as the basis of OSHA policy.)
NMEA: The LD50 for NMEA is 90 mg/kg
following administration by esophageal probe to the rat. In a
chronic effects study, 4 rats received 2 mg/kg and 11 rats 1 mg/kg
daily in the drinking water. Five of the rats in the second group
died without tumors and all the other rats had extensive liver
cancers. One animal had a tumor of the vagina. The mean carcinogenic
doses were 750 and 420 mg/kg and the mean induction periods were 360
and 500 days respectively. The substance was designated a weaker
carcinogen than N-nitrosodiethylamine (Ref. 5.8.).
NDiPA: The LD50 for NDiPA was 850 mg/kg
following oral administration to the rat. In a chronic effects
study, 25 and 50 mg/kg of NDiPA were administered to 2 groups of
rats daily in the drinking water. The experiment was discontinued
after a total dose of 11 and 14 g/kg had been administered. These
rather large doses produced liver cancer in only 9 of a total of 20
test animals. The mean induction periods were 770 and 430 days for
each group respectively. The weak carcinogenic effect of NDiPA was
attributed to steric hindrance of the enzymatic hydroxylation of the
alpha-carbon atom due to the branched alkyl groups
(Ref. 5.8.). In another chronic effects study, 450 and 2,400 mg of
NDiPA were administered to 2 groups of rats over 50 and 40 weeks.
Twenty-two of thirty rats had tumors primarily of the
nasal turbinate (Ref. 5.36.).
NMBA: The LD50 for NMBA is 130 mg/kg
following oral administration to the rat. The lowest dose which
resulted in liver tumors for 50% of the test rats was 600 mg/kg
NMBA. The substance was administered by intraperitoneal injection.
(Ref. 5.37.). Inhalation of NMBA has been reported to result in
incidences of 100% for esophageal tumors and 23% for nasal tumors in
rats (Ref. 5.38. and 5.39.).
NEBA: The LD50 for NEBA was 380 mg/kg,
following oral administration to the rat. In a chronic effects
study, five rats received 10 mg/kg and 25 rats 5 mg/kg NEBA daily in
the drinking water. All of the test animals died between the 175th
and the 290th day with extensive cancer of the esophagus. Three
animals also had liver cancer and one a pulmonary cancer. The mean
carcinogenic doses were 1.6 and 0.97 g/kg and the mean induction
periods were 200 and 240 days respectively. In another experiment,
15 rats received 25 mg/kg once per week by intravenous injections.
Five animals developed papillomas and nine cancer of the esophagus.
Six rats had liver tumors, one cancer of the ethmoturbinalia and
another cancer of the pancreas. Seven animals had tumors in multiple
organs. The mean carcinogenic dose was 1.04 g/kg and mean induction
period was 363 days. Bladder cancer which is often the result of
exposure to N-nitrosodibutylamine was not observed in
these experiments (Ref. 5.8.).
NPBA: The daily oral administration of 415 mg NPBA per animal, in
the drinking water, for 16 to 19 weeks led to a 100% incidence of
liver cancer in the rat. Six of 10 rats also developed esophageal
papillomas (Ref. 5.40.).
NDAmA: The LD50 for NDAmA was 3000
mg/kg following subcutaneous administration to the rat. The oral
administration of 100 mg/kg NDAmA, daily, in the diet led to liver
cancer in 17 of 18 animals; in 5 animals the liver tumors were
accompanied by pulmonary metastases. One animal also had lung
cancer. The carcinogenic mean dose was 48 g/kg and the mean
induction period was 360 days. In another chronic experiment, 500
mg/kg of undiluted NDAmA were administered to rats once per week by
subcutaneous injection. Only one animal developed liver cancer but
six of ten rats died with lung cancer. The mean carcinogenic dose
was 12 g/kg and the mean induction period was 340 days. This
experiment was seen as fundamentally important because it
demonstrated that pulmonary cancer is not only produced by locally
acting inhaled agents but also that it can result from absorbed
carcinogens. These experiments also show that the affected organs
are not only a function of the chemical and physical properties of
the substance but also by the size of the dose and mode of
administration (Ref. 5.8.).
Molecular Structure and carcinogenicity: A few generalities
regarding the carcinogenic properties of nitrosodialkylamines have
been made. Branching of the alkyl group at the alpha carbon tends to
decrease carcinogenicity. Increasing molecular weight and polarity
seems also to reduce carcinogenic activity. The carcinogenicity of
unsymmetrical nitrosamines is often selective toward the esophagus
(Ref. 5.9.).
4.10. Reproducibility
Six vapor spiked ThermoSorb/N tubes and a draft copy of this
procedure were given to a chemist unassociated with this evaluation.
The samples were analyzed after one day of storage at ambient
temperature. The recoveries and standard deviations are presented in
Table 4.10. The samples were spiked at the target concentrations.
Table 4.10. Reproducibility Study
|
|
NMEA |
NDiPA |
NMBA |
NEBA |
NPBA |
NDAmA |
|
amount vapor spiked, µg
recovery,
%
, % SD, % |
0.20
100.0 100.0 110.0 100.0 105.0 90.0
100.8 6.65 |
0.22
100.0 95.5 104.5 100.0 104.5 90.9
99.2 5.29 |
0.19
100.0 94.7 105.3 100.0 105.3 100.0
100.9 3.99 |
0.22
100.0 95.5 104.5 100.0 100.0 100.0
100.0 2.85 |
0.20
100.0 110.0 105.0 105.0 100.5 100.0
103.4 4.01 |
0.30
106.7 100.0 110.0 103.3 103.3 103.3
104.4 3.45 |
|
Figure 1. The metabolic activation of
N-nitrosodialkylamines.
Figure 4.1. The detection limits of the analytical
procedure.
Figure 4.4.1. N-nitrosomethylethylamine calibration
curve.
Figure 4.4.2. N-nitrosodiisopropylamine calibration
curve.
Figure 4.4.3. N-nitrosomethylbutylamine calibration
curve.
Figure 4.4.4. N-nitrosoethylbutylamine calibration
curve.
Figure 4.4.5. N-nitrosopropylbutylamine calibration
curve.
Figure 4.4.6. N-nitrosodiamylamine calibration
curve.
Figure 4.5.1. Breakthrough study one.
Figure 4.5.2. Breakthrough study two.
Figure 4.7.1. Ambient temperature storage test for
nitrosomethylethylamine.
Figure 4.7.2. Ambient temperature storage test for
N-nitrosodiisopropylamine.
Figure 4.7.3. Ambient temperature storage test for
N-nitrosomethylbutylamine.
Figure 4.7.4. Ambient temperature storage test for
N-nitrosoethylbutylamine.
Figure 4.7.5. Ambient temperature storage test for
N-nitrosopropylbutylamine.
Figure 4.7.6. Ambient temperature storage test for
N-nitrosodiamylamine.
Figure 4.7.7. Reduced temperature storage test for
N-nitrosomethylethylamine.
Figure 4.7.8. Reduced temperature storage test for
N-nitrosodiisopropylamine.
Figure 4.7.9. Reduced temperature storage test for
N-nitrosomethylbutylamine.
Figure 4.7.10. Reduced temperature storage test for
N-nitrosoethylbutylamine.
Figure 4.7.11. Reduced temperature storage test for
N-nitrosopropylbutylamine.
Figure 4.7.12. Reduced temperature storage test for
N-nitrosodiamylamine.
Figure 4.8.1. GC/TRA chromatogram of the analytes with the Carbowax
20M column. The column temperature was programmed from 150 to 220°C at
4°C/min. The injector was set at 150°C and the carrier gas flow rate was
30 mL/min.
Figure 4.8.2. GC/TEA chromatogram of a mixture of
N-nitrosamines with the Carbowax 20 M column. The column
temperature was programmed from 150 to 220°C at 4°C/min. The injector
was set at 150°C and the carrier gas flow rate was 30 mL/min.
Figure 4.8.3. GG/TEA chromatogram of a mixture of
N-nitrosamines with the Versamid 900 column. The column
temperature was programmed from 150 to 220°C at 4°C/min. The injector
was set at 150°C and the carrier gas flow rate was 30 mL/min.
Figure 4.8.4. HPLC/TEA chromatogram of a mixture of
N-nitrosamines with the CN column. The mobile phase was
94.5% 2,2,4-trimethylpentane, 5% dichloromethane and 0.5%
isopropanol. The flowrate was 1.3 mL/min.
5. References
5.1. Hendricks, W. Volatile Nitrosamine Mixture I (Method 27,
Organic Methods Evaluation Branch, OSHA Analytical Laboratory, Salt
Lake City, Utah) Unpublished (2-81).
5.2. Fine, D.H.; Rounbehler, D.P.; Sawicki, E. and Krost, K.
Environ. Science Technol., 11, 577-580, (1977).
5.3. Fine, D.H. Oncology, 37, 199-202, (1980).
5.4. Rounbehler, D.P.; Reisch, J.W.; Coombs, J.R. and Fine, D.H.
Anal. Chem., 52, 273-276, (1980).
5.5. Fisher, R.L.; Rieser, R.W., and Lasoski, B.A. Anal.
Chem., 49, 1821-1823, (1977).
5.6. Sen, N.P. "Environmental Carcinogens Selected Methods of
Analysis, Volume 1-Analysis of Volatile Nitrosamines in
Food", IARC Scientific Publications No. 18, Egan, H.,
Editor-in-Chief, International Agency for Research on
Cancer: Lyon (1978).
5.7. Instruction Manual - Thermal Energy Analyzer, Model 502/LC,
Thermal Electron Corporation, Waltham, Mass. 02145, 3-1,
(12-77).
5.8. Druckrey, D.; Preussmann, R.; Ivankovic, S. and Schmahl, D.
Z. Krebsforsch, 69, 103-201, (1967).
5.9. Edelman, A.S.; Kraft, P.L.; Rand, W.M., and Wishnok, J.S.
Chem. Biol. Interactions, 31, 81-92, (1980).
5.10. McCann, J.; Choi, E.; Yamasaki, E., and Ames, B.N. Proc.
Nat. Acad. Sci. USA, 72, 5135-5139, (1975).
5.11. Yahagi, T.; Nagao, M.; Seino, Y.; Matsushima, T.; Sugimura,
T., and Okada, M. Mutation Res., 48, 121-130,
(1977).
5.12. Ember, L.R. Chem. Eng. News, 58, 20-26,
(1980).
5.13. Rounbehler, D.P.; Krull, I.S.; Goff, E.U.; Mills, K.M.;
Edwards, G.S.; Fine, D.H.; Rheinhold, V.; Morrison, J.; Fagen, J.M.
and Carson, G.A. Fd. Cosmet. Toxicol. 17, 487-491,
(1979).
5.14. Fajen, J.M.; Carson, G.A.; Rounbehler, D.P.; Fan, T.Y.; Vita,
R.; Goff, V.E.; Wolf, M.H.; Edwards, G.S.; Fine, D.H.; Reinhold, V.
and Biemann, K. Science, 205, 1262-1264, (1979).
5.15. Chem. Eng. News, 57, 6, (1979).
5.16. Chem. Eng. News, 57, 18, (1979).
5.17. Brunnemann, K.D.; Yu, L. and Hoffmann, D. Cancer
Research, 37, 3218-3222, (1977).
5.18. Day, E.W.; West, S.D.; Koenig, D.K. and Powers, F.L. J.
Agric. Food Chem., 27, 1081-1085, (1979).
5.19. Fiddler, W.; Pensabene, J.W.; Doerr, R.C. and Dooley, C.J.
Fd. Cosmet. Toxicol., 15, 441-443, (1977).
5.20. Spiegelhalder, B.; Eisenbrand, G. and Preussmann, R.
Angew. Chem. Int. Ed. Engl., 17, 367-368, (1978).
5.21. Archer, M.C. and Wishnok, J.S., J. Environ. Sci.
Health, 10 & 11, 583-590, (1976).
5.22. Goff, E.U.; Coombs, J.R. and Fine, D.H. Anal. Chem.,
52, 1833-1836, (1980).
5.23. Challis, B.C.; Edwards, A.; Hunna, R.R.; Kyrtopoulos, S.A.
and Outram, J.P. "Environmental Aspects of N-Nitroso
Compounds, IARC Scientific Publications No. 19", Walker, E.A., Lyle,
R.E., Castegnaro M. and Griciute, L. Eds., International Agency for
Research on Cancer: Lyon, 127-139, (1978).
5.24. March, J. "Advanced Organic Chemistry: Reactions, Mechanisms
and Structure", McGraw-Hill, Inc.: New York,
430-431, (1968).
5.25. Fine, D.H. "Monitoring Toxic Substances", Schuetzle, D. Ed.,
American Chemical Society Symposium, Series 94: Washington, D.C.,
246-254, (1979).
5.26. Registry of Toxic Effects of Chemical Substances, 1979
Edition. (Lewis, R.J. and Tatken, R.L., Eds.) U.S. Department of
Health, Education and Welfare, Public Health Service, Center for
Disease Control, National Institute for Occupational Safety and
Health, U.S. Government Printing Office, Washington, D.C. (1980).
5.27. "CRC Handbook of Chemistry and Physics", CRC Press: Boca
Raton, FL (1979).
5.28. "IARC Monographs on the Evaluation of the Carcinogenic Risk
of Chemicals to Humans, Some N-nitroso Compounds, Volume
17", International Agency for Research on Cancer: Lyon (1978).
5.29. Wishnok, J.S. and Tannenbaum, S.R. Anal. Chem., 49,
715A-718A, (1977).
5.30. Lijinsky, W. and Epstein, 5.5. Nature, 225,
21-23, (1970).
5.31. Sander, J.; Schweinsberg, F.; La Bar, J. and Burkle, G. "GANN
Monograph on Cancer Research 17", 145-160, (1975).
5.32. Iqbal, Z.M.; Dahl, K. and Epstein, 5.5. Science, 207,
1475-1476, (1980).
5.33. Wang, T.; Kakizoe, T.; Dion, P.; Furrer, R.; Varghese, A.J.
and Bruce, W.R. Nature, 276, 280-281, (1978).
5.34. Kakizoe, T.; Wang, T.; Eng, V.W.S.; Furrer, R.; Dion, P. and
Bruce, W.R. Cancer Research, 39, 829-832, (1979).
5.35. "ThermoSorb/N Air Sampler-Instructions for
Monitoring", 5-25 Thermo Electron Corporation (1980).
5.36. Lijinsky, W. and Taylor, W. J. Natl. Cancer Inst., 62,
407-410, (1979).
5.37. Heath, D.F. and Magee, P.N. Brit. J. Indur. Med., 19,
276-282, (1962).
5.38. Druckrey, H.; Landschutz, C. and Preussmann, R. Z.
Krebsforsch, 75, 221-224, (1971).
5.39. Druckrey, H. and Landschutz, C. Z. Krebsforsch, 75,
221-224, (1971).
5.40. Okada, M.; Suzuki, E. and Hashimoto, Y. Gann, 67,
825-834, (1976).
5.41. Pitts, J.N.; Grosjean, D.; Cauwenberghe, K.V.; Schmid, J.P.
and Fitz, D.R. Environ. Science Technol., 12,
946-953, (1978).
|