DIMETHYLNITROSAMINE
Method no.: |
06 |
|
Matrix: |
Air |
|
OSHA Standard: |
OSHA regulated carcinogen. The standard applies to
all solid or liquid mixtures that contain more than 1.0%
dimethylnitrosamine by weight or volume. |
|
Target concentration: |
6.4 µg/m3 (2.1 ppb) |
|
Procedure: |
Collection on treated Florisil adsorbent tubes. Two
tubes are used in series, each of which has been pretreated with 10
mg DL-a-tocopherol. Samples are desorbed
with a 50/50 (v/v) methylene chloride-methyl alcohol solution.
Analysis is by gas chromatography with chemiluminescence
detection. |
|
Detection limit based on recommended air
volume: |
0.4 µg/m3 (130 ppt) (For
analytical method only) |
|
Recommended air volume and sampling rate: |
25 L at 0.2 L/min |
|
Standard error of estimate at the target
concentration: (Section 4.3.) |
7.5% |
|
Special requirements: |
The treated Florisil tubes must be protected from
light during and after sampling. |
|
Status of method: |
A sampling and analytical method which has been
subjected to the established evaluation procedures of the Organic
Methods Evaluation Branch. |
|
Date: May 1979 |
Chemist: Warren
Hendricks |
Organic Methods Evaluation Branch OSHA Analytical
Laboratory Salt Lake City, Utah
1. General Discussion
1.1. Background
1.1.1. History
Sample Collection: Dimethylnitrosamine (DMN) vapors have
been collected using cryogenic techniques that involve the use of
successive cold traps (Ref. 5.1.), ambient temperature KOH bubblers
(Ref. 5.2.), Tenax GC cartridges (Ref. 5.3.), and XAD-4 resin tubes
(Ref. 5.4.). For various reasons, these air sampling procedures are
not well suited for use by OSHA personnel. Therefore, the primary
emphasis of this work has been to develop new air sampling
techniques.
Analytical: The analytical procedures for airborne DMN
usually include separation by gas chromatography and detection by
one or more of the following techniques: mass spectrometer (Ref.
5.5.), Coulson Electrolytic Conductivity Detector (Ref. 5.6.), Hall
Electrolytic Conductivity Detector (Ref. 5.7.), nitrogen selective
alkali flame-ionization detector (Ref. 5.8.) and Thermal Energy
Analysis (Ref. 5.9.). This method utilizes separation by gas
chromatography and detection by the Thermal Energy Analyzer (TEA).
The TEA detector was selected because it is both sensitive and
selective for nitrosamines.
1.1.2. Toxic effects (This section is for information only and
should not be taken as a basis for OSHA policy.)
Acute: The LD50,
in the rat, for DMN is 26 mg/kg and the
LC50 is 78 ppm over 4 h (Ref. 5.10.). A
single dose of about 25 mg/kg DMN administered orally, to the rat,
or by intravenous, intraperitoneal or subcutaneous injection
produces serious destruction of liver tissue accompanied by
hemorrhages into the liver and lungs. Often there occurs a serious
accumulation of fluid in the abdominal area and blood in the lumen
of the intestines. Death usually occurs in 2-4 days or the animal
recovers completely. Rabbits, mice, guinea pigs, and dogs all
develop similar liver damage (Ref. 5.11).
Chronic: When DMN was administered, to the rat, in
continuous doses low enough for the animal to survive 30 weeks or
longer, the result was cancer of the liver. If the agent was given
at higher concentrations for shorter periods, or as a single dose,
kidney tumors developed. It has been shown that liver and kidney
tumors can infrequently develop simultaneously after exposure to
DMN. However, in general, continuous low exposures cause cancer of
the liver and higher concentrations for short periods (or as a
single dose) result in kidney tumors (Ref. 5.11.).
Although, in the rat, cancers of the liver and the kidney are the
most important result of exposure to DMN, the agent will also
produce lung and nasal sinus tumors in the animal. DMN will cause
tumors of the liver, kidney, and lung in the mouse, of the liver in
the Syrian hamster and of the liver in the Rainbow trout (Ref.
5.11.).
The fact that a single exposure to DMN can result in cancer has
tremendous implications with respect to man. While there is no
direct evidence that exposure to DMN leads to cancer in humans,
indirect evidence, obtained from laboratory experiments that
measured the relative metabolic rates of DMN by rat and human liver
slices, indicate that man is probably about as sensitive to the
carcinogenic action of DMN as is the rat (Ref. 5.12.).
1.1.3. Workplace exposure
It is more difficult to determine point sources for DMN emissions
than for other toxic agents because DMN is not extensively used by
industry and most current occupational exposures will probably occur
as a result of the chemical formation of the compound from its
precursors rather than from the known utilization of the chemical.
The chemical reaction, in the condensed phase, between nitrous
acid and dimethylamine (DMA) or trimethylamine (TMA) to form DMN is
well known (Ref. 5.13.). Recently it has been shown that DMA and TMA
can react with oxides of nitrogen in the vapor phase to give DMN as
a reaction product (Ref. 5.14.). This means that even though DMN is
not used at a particular location, it may be formed from its
precursors and therefore be found in the occupational environment.
The fact that oxides of nitrogen can chemically react with amines
to produce airborne nitrosamines is of special interest in light of
the fact that there appears to be a statistical correlation between
high concentrations of NO2 and high
incidence of cancer in some urban areas (Ref. 5.15.).
NO2, in itself, is probably not a
carcinogen (Ref. 5.16.).
Exposure to DMN can occur during operations in which DMA or TMA
is utilized or produced. The exposure results because both DMA and
TMA are often contaminated with the nitrosamine. Further, if the
amine is used as a chemical intermediate, DMN can possibly appear in
the reaction product (Ref. 5.17.).
DMN is used extensively in cancer research facilities. Human
exposure occurs when unchanged DMN is excreted by the laboratory
animals (Ref.5.7.).
DMN has been used as an industrial solvent and as a chemical
intermediate in the production of
1,1-dimethylhydrazine. Other uses or proposed uses
include: as a nematocide, as a lubricant additive, as an
antioxidant, as a softener for copolymers and in electrical
condensers to increase the dielectric constant (Ref. 5.18.).
Non-occupational exposure to DMN can occur through the use of
nitrite cured food products. DMA has been shown to react with
nitrite to produce DMN in the stomachs of rats (Ref. 5.18.). DMN has
been reported to be a component of tobacco and tobacco smoke and to
be present in some alcoholic beverages (Ref. 5.19.).
1.1.4. Physical properties - (Ref. 5.18. and 5.20.)
CAS no.: |
62-75-9 |
mol wt: |
74.1 |
appearance: |
nonviscous yellow liquid |
boiling point: |
149-150°C (755 mm Hg), 50-52°C (14 mm Hg) |
density: |
1.0048 (20/4°C) |
refract. index: |
1.4368 (25°C/D) |
absorp. spec.: |
230 nm log e
3.86 |
(in water) |
332 nm log e
1.98 |
solubility: |
miscible with water in all proportions, soluble
in all common organic solvents, |
volatility: |
very volatile |
molecular structure: |
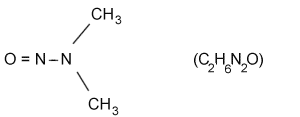 |
synonyms: |
Dimethylamine, N-nitroso-;
N,N-dimethylnitrosamine; DMN; DMNA;
dimethylnitramine; nitrous dimethylamide and
N,N-dimethylnitrosoamine. |
1.2. Detection limit, precision, sensitivity and working range
1.2.1. The detection limit for the analytical procedure is 50 pg.
The coefficient of variation was 0.15 at this concentration (Section
4.1.). The detection limit was determined using 5-µL injections from
standard solutions.
1.2.2. The pooled coefficient of variation for the analytical
procedure over the range of 80 to 320 ng per sample was 0.042
(Section 4.2.). This represents an air concentration range of from
3.2 to 12.8 µg/m3 based on the recommended
sampling and analytical procedures.
1.2.3. The sensitivity of the analytical procedure over a
concentration range of 80 to 320 ng (3.2 to 12.8
µg/m3 based upon the recommended air
sampling volume of 25 L) is 227908 area units (HP-5840A) per µg/mL.
The sensitivity is determined by the slope of the calibration curve
(Section 4.2.). The sensitivity will vary somewhat with the
particular instrumentation used in the analysis.
1.2.4. The lower limit of the estimated working range, assuming
adequate desorption efficiency, is 0.4
µg/m3. The upper limit of the working
range is dependent on the capacity of the treated Florisil tubes.
1.3. Accuracy
1.3.1. The overall procedure must provide results that are within
±25% of the true value at the 95% confidence interval.
1.3.2. The recovery of analyte from the collection medium during
storage must be 75% or greater.
1.3.3. The overall procedure has met the above validation
criteria (Section 4.3.).
1.4. Advantages
1.4.1. The sampling procedure is convenient.
1.4.2. The significance of artifactual formation of DMN upon the
sampling device has been eliminated through pretreatment of the air
sampler.
1.4.3. The analytical procedure is quick, sensitive, and
reproducible.
1.4.4. Reanalysis of the samples is possible.
1.4.5. The samples are stable, even when stored at room
temperature for 17 days.
1.4.6. It may be possible to determine other nitrosamines
simultaneously.
1.4.7. The effects of potential interferences are reduced through
the use of a selective detector (the TEA) and can be further reduced
by proper selection of GC parameters.
1.5. Disadvantages
1.5.1. The relative humidity of the sampled air affects the
ability of the adsorbent to retain the analyte.
1.5.2. The sampling method has not been field tested.
2. Sampling Procedure
2.1. Apparatus
2.1.1. An approved and calibrated personal sampling pump whose
flow can be determined to ±5% at the recommended flow rate.
2.1.2. Florisil adsorbent tubes: Glass tubes, 6-mm o.d., 4-mm
i.d., 7-cm length, containing 100-mg front and 50-mg rear (separated
by a 2-mm portion of urethane foam or silylated glass wool) sections
of 20/40 mesh Florisil. SKC Inc. Catalog No. 226-39 or equivalent.
Each tube is pretreated with 10 mg of DL-a-tocopherol (Section 4.4.).
2.2. Reagents
None required.
2.3. Sampling Technique
2.3.1. The air sampler is composed of two treated Florisil tubes
in series. The tubes are easily connected with an end cap that has
been modified by cutting off the closed portion.
2.3.2. Connect the air sampler to the sampling pump with flexible
tubing. The 50-mg section of each tube should be positioned toward
the sampling pump. Cover each tube of the air sampler with masking
tape or other suitable material to prevent light from reaching the
adsorbent.
2.3.3. The air sampler should be placed in a vertical position
during sampling to minimize channeling.
2.3.4. Sampled air should not pass through any hose or tubing
before entering the sampling device.
2.3.5. Immediately after sampling, separate the air sampler into
its component tubes, identify each tube as front or backup and seal
each tube with plastic end caps. Also, wrap each sample end to end
with official OSHA seals.
2.3.6. With each batch of samples, submit at least one blank tube
from the same lot used for samples. This tube should be subjected to
exactly the same handling as the samples (seal, transport) except
that no air is drawn through it.
2.3.7. Transport the samples (and corresponding paperwork) to the
lab for analysis.
2.3.8. If bulk samples are submitted for analysis, they should be
transported in glass containers with Teflon-lined caps. The samples
must be protected from light. These samples must not be put in the
same container used for the treated Florisil tubes.
2.4. Breakthrough
The relative humidity of the sampled air has a significant effect
on the ability of the Florisil adsorbent to retain DMN. For example,
after more than 1000 L of air at about 30% relative humidity were
drawn through a treated Florisil tube containing 0.6 µg DMN (added via
liquid injection), all the analyte remained on the front section of
the single tube. In contrast, when 60 L of air at near 100% relative
humidity were drawn through a tube containing a similar amount of DMN,
no analyte was retained on either section of the tube.
The analyte was found to migrate from the front to the rear section
of a treated Florisil tube. The migration was complete after three
days storage at room temperature with the DMN evenly distributed
throughout the adsorbent.
Because of the humidity effect upon DMN retention and also because
of the ability of the analyte to migrate from the front to the rear
section of the tube, it was decided to use two treated Florisil tubes
in series.
Laboratory experiments indicate that 25 L of air at 80% relative
humidity and 22°C may be sampled with no loss of analyte. When the air
sampler was challenged with 0.32 µg DMN, as a vapor, breakthrough from
the front to the rear tube, at this humidity was about 30%.
No quantitative breakthrough information can be obtained from the
analysis of individual tubes from a particular air sampler, but, if
the amount found on the rear tube is 45%, or greater, of the total,
then it is possible that some of the analyte may have been lost.
2.5. Desorption efficiency
2.5.1. The average desorption from DL-a-tocopherol treated Florisil adsorbent
tubes spiked with 80, 160, and 320 ng DMN was 87.8% (Section 4.5.).
The spiked tubes represent an air concentration range of 3.2 to 12.8
µg/m3 based upon the recommended air
volume.
2.5.2. The desorption efficiency for DMN may vary from one
laboratory to another and also from one lot of DL-a-tocopherol treated Florisil tubes to
another. Therefore, it is necessary for each laboratory to determine
the desorption efficiency for each lot of DL-a-tocopherol treated Florisil tubes.
2.6. Recommended air volume and sampling rate
2.6.1. The recommended air volume is 25 L.
2.6.2. The recommended sampling rate is 0.2 L/min.
2.7. Interferences
2.7.1. Since it is possible that the precursors of DMN, such as
DMA, TMA, and various nitrosating agents (oxides of nitrogen,
nitrites, etc.), are present in the environment, it is conceivable
that DMN may be formed upon the sampling device and not be present
in the sampled air.
Laboratory experiments indicate that it is possible to form DMN
from its precursors on untreated Florisil tubes. Further experiments
show that when the Florisil tube is treated with 10 mg of
DL-a-tocopherol, the formation
of DMN does not take place.
2.7.2. At the present time, it is unknown if any compound would
severely interfere with the collection of DMN on treated Florisil
tubes. In general, the presence of other compounds will reduce the
breakthrough volume for a particular compound.
2.7.3. Any compound which is suspected of interfering with the
collection or analysis should be listed on the sampling data sheet.
2.7.4. Light will decompose DMN (Ref. 5.18.). The air sampler
must be protected from light during and after sampling.
2.8. Safety precautions (sampling)
2.8.1. Observe due care when working with the sharp ends of the
air sampler.
2.8.2. Attach the sampling equipment to the worker in such a
manner that it will not interfere with work performance.
2.8.3. Follow all safety practices that apply to the work area
being sampled.
3. Analytical Method
3.1. Apparatus
3.1.1. A gas chromatograph interfaced to a Thermal Energy
Analyzer.
3.1.2. A GC column capable of resolving DMN from the desorption
solvent and potential interferences. The column used in this work
was a 12-ft × 1/8-in. stainless steel column containing 10% Carbowax
20M with TPA on 80/100 mesh Chromosorb W AW.
3.1.3. An electronic integrator or other suitable method to
measure peak area.
3.1.4. An analytical balance capable of accurately weighing
standards.
3.1.5. Vials. 2-mL vials with Teflon-lined caps.
3.1.6. Microliter syringes. 5-µL syringe for sample injections,
and convenient sizes.
3.1.7. Pipets. Convenient sizes for diluting standards. A
1-mL pipet for desorbing solvent.
3.1.8. Volumetric flasks. Convenient sizes for diluting
standards.
3.1.9. Dewar flask. Convenient sizes for liquid nitrogen.
3.2. Reagents
3.2.1. DMN, authentic primary standard, 98% minimum.
3.2.2. Methyl alcohol, chromatographic grade.
3.2.3. Methylene chloride, chromatographic grade.
3.2.4. Isopropyl alcohol, chromatographic grade.
3.2.5. Ethyl alcohol, U.S.P., 95%.
3.2.6. Gases, purified GC grade, helium and medical grade oxygen.
3.2.7. Nitrogen, liquid.
3.3. Sample Preparation
3.3.1. The status of the OSHA seal on each sample is noted and
recorded as intact, broken, or none.
3.3.2. The field and laboratory identification numbers on the
sample are checked against those on the sample identification
sheets.
3.3.3. Suitable precautions must be undertaken to prevent
exposure of samples to light. DMN will photodecompose easily.
3.3.4. The front and rear tubes from each sampler are transferred
to separate 2-mL vials.
3.3.5. Each tube is desorbed with 1.0 mL of desorbing solution.
The desorbing solution is composed of equal parts by volume methyl
alcohol and methylene chloride.
3.3.6. The vials are sealed immediately with Teflon-lined caps
and are desorbed for 30 min with intermittent shaking.
3.4. Standard preparation
3.4.1. Stock standards are prepared by diluting a weighed amount
of DMN with isopropyl alcohol. The stock standard is diluted to the
working range with isopropyl alcohol (See Section 3.8. Safety
Precautions).
3.4.2. A solution composed of 0.14 µg/mL DMN in isopropyl alcohol
equals an air concentration of 6.4 µg/m3
for a 25-L air sample desorbed with 1.0 mL of desorbing solution.
This amount is corrected for the desorption efficiency.
3.4.3. Standards are stored in dark bottles under refrigeration.
3.5. Analysis
3.5.1. GC conditions
helium (carrier gas) flow rate: |
30 mL/min |
injector temperature: |
200°C |
column temperature: |
170°C |
TEA transfer line temperature: |
205°C |
injection volume: |
5 µL |
elution time: |
2 min |
3.5.2. TEA conditions
oxygen pressure: |
9 PSI |
CC pyrolyzer furnace temperature: |
475°C |
chamber vacuum: |
1.8 mm Hg |
coarse zero: |
high |
calibrate: |
0.0 |
at tenuator: |
1 |
cold trap temperature: |
-130°C (ethyl alcohol and liquid
nitrogen) |
Complete instructions for the TEA are found in its manual.
3.5.3. Chromatogram (Section 4.6.)
3.5.4. Peak areas are measured by an electronic integrator or
other suitable means.
3.5.5. An external standard procedure is used to prepare a
calibration curve from the analysis of at least three different
standard solutions. The calibration curve is prepared daily. The
integrator is calibrated to report results in µg/mL after correction
for desorption efficiency.
3.5.6. Bracket the samples with analytical standards.
3.6. Interferences
3.6.1. Any compound that has the same GC retention as DMN and
will elicit a response from the TEA detector is an interference.
3.6.2. GC parameters may be changed to circumvent most
interferences.
3.6.3. Retention time on a single GC column is not proof of
chemical identity. Samples should be confirmed by GC/MS or other
suitable means when required.
3.7. Calculations
3.7.1. The integrator value in µg/mL (corrected for desorption
efficiency) is used for reference only. More reliable results are
obtained by use of the calibration curve. The peak area for each
standard is compared to its concentration in µg/mL (corrected for
desorption efficiency) and the equation for the best straight line
through the data points is determined by linear regression.
3.7.2. The concentration in µg/mL (corrected for desorption
efficiency) for a sample, is determined by comparing the area of a
particular sample to the calibration curve.
3.7.3. Analytical results from the two tubes that compose a
particular air sampler are added together.
3.7.4. The air concentration for a sample is calculated by the
following equation:
DMN, µg/m3 = (A)(B)(1000)/C
where |
A |
= |
µg/mL from 3.7.3. |
|
B |
= |
desorption volume |
|
C |
= |
air volume in
liters |
3.7.5. To convert µg/m3 to parts per
billion (ppb) the following relationship is used:
DMN, ppb =
(µg/m3)(24.46)/74.1
where |
µg/m3 |
= |
result from 3.7.4. |
|
24.46 |
= |
molar volume at 25°C and 760 mm Hg. |
|
74.1 |
= |
molecular weight of
DMN |
3.8. Safety precautions (analytical)
3.8.1. DMN is an extremely potent carcinogen and utmost care must
be exercised when working with this compound.
3.8.2. Avoid skin contact with liquid nitrogen and the solvents.
3.8.3. Confine the use of solvents to a fume hood.
3.8.4. Wear safety glasses in all laboratory areas.
3.8.5. Check to be sure that the TEA exhaust is connected to a
fume hood.
4. Backup Data
4.1. Detection limit
The following data were generated by replicate 5-µL injections of a
standard solution whose concentration was 0.01 µg/mL.
The detection limit was determined to be 50 pg which is equivalent
to 0.4 µg/m3 based on the recommended air
volume.
Peak heights were used because integrated area data were unreliable
at this level.
Table 4.1. Detection Limit Data
|
injection |
peak height, mm |
statistics |
|
1 |
98 |
|
2 |
89 |
|
= |
85.17 |
3 |
95 |
SD |
= |
12.58 |
4 |
70 |
CV |
= |
0.15 |
5 |
69 |
|
6 |
90 |
|
|
4.2. Instrument response to DMN and Precision
The data in Table 4.2. represent multiple injections of standard
solutions. The injection volume was 5 µL and the concentrations of the
standards were 0.08 µg/mL, 0.16 µg/mL and 0.32 µg/mL. Peaks were
integrated by a Hewlett-Packard 5840A Gas Chromatograph.
Table 4.2. Instrument Response and Precision
|
× target conc. |
0.5× |
1× |
2× |
pg/injection |
400 |
800 |
1600 |
|
area |
20550 |
41100 |
76440 |
counts |
18370 |
38470 |
74160 |
|
19600 |
39830 |
79200 |
|
20480 |
42950 |
73200 |
|
19440 |
42310 |
74880 |
|
20460 |
38400 |
72160 |
|
 |
19816.7 |
40510.0 |
75006.7 |
SD |
857.1 |
1929.6 |
2519.5 |
CV |
0.0433 |
0.0476 |
0.0336 |
|
= 0.042 |
|
|
A typical calibration curve is shown in Figure 4.2. The curve
indicates a linear relationship and has a slope of 227908 area counts
per (µg/mL).
4.3 Storage
Samples were generated by the liquid injection of DMN on Tenax GC
tubes containing about 45 mg Tenax GC. The tubes were allowed to
equilibrate overnight and then were placed in front of the treated
Florisil tubes. Twenty-five liters of humid air, at 80% relative
humidity and 22°C, were drawn through the sampling train. The DMN on
the Tenax GC tube was desorbed by the humid air and was deposited on
the air sampler. Studies conducted at ambient relative humidity and
temperature indicated that desorption was essentially complete after 5
L of air had passed through the Tenax GC tube (Figure 4.3.1.). In
these studies the spiked Tenax GC tube was connected directly to the
TEA.
The following data represents the effects of storage at ambient
(21-26°C) temperature on DMN collected on treated
Florisil tubes. The recoveries are not corrected for desorption
efficiency. A graphical representation of the data may be found in
Figure 4.3.2. A storage study at reduced temperature was not
performed.
Table 4.3. Storage Test at Ambient Temperature
|
storage time |
% recovery |
(days) |
|
|
0 |
87.5 |
84.4 |
82.5 |
3 |
87.5 |
80.0 |
80.0 |
6 |
85.0 |
88.8 |
97.5 |
10 |
78.8 |
85.6 |
87.5 |
13 |
80.6 |
78.1 |
78.1 |
17 |
81.9 |
94.4 |
83.8 |
|
4.4. Preparation of pretreated sampling tubes
4.4.1 Reagents
Methylene chloride, chromatographic grade.
DL-a-tocopherol, 90%
minimum - available from ICN Pharmaceutical Inc., K & K Labs
Division, Life Sciences Group (1979 Catalog No. 18787). Prepare a
solution containing 50 mg/mL in methylene chloride. Store the
solution under refrigeration in a dark bottle.
4.4.2. Procedure
Each tube is pretreated with 10 mg of DL-a-tocopherol. This is accomplished by
first breaking open both ends of each tube and adding 200 µL of the
DL-a-tocopherol solution. Both
sections of the tube should be uniformly wet by the solution. The
methylene chloride is allowed to evaporate in a fume hood overnight
and then both ends of the tubes are sealed with the plastic end caps
supplied with the tubes. The tubes should be stored in a freezer
with suitable precautions taken to prevent their contamination. The
tubes are stable, when stored in a freezer, for at least a month.
4.5. Desorption
Samples representing 3.2, 6.4, and 12.8
µg/m3 based on 25-L air volumes were
prepared by injecting liquid standards on treated Florisil tubes.
Table 4.5. Desorption Efficiency
|
|
µg/m3 |
3.2 |
6.4 |
12.8 |
|
desorption |
70.0 |
90.0 |
83.8 |
efficiency, |
77.5 |
86.9 |
83.8 |
% |
68.6 |
78.8 |
87.5 |
|
130.0 |
74.4 |
82.2 |
|
127.5 |
91.9 |
86.6 |
|
75.0 |
100.6 |
85.6 |
|
 |
91.4 |
87.1 |
84.9 |
|
overall average =
87.8 |
|
4.6. Chromatogram
A typical chromatogram is shown in Figure 4.6.
Figure 4.2. Dimethylnitrosamine calibration curve.
Figure 4.3.1. The rate at which dimethylnitrosamine is
desorbed from Tenax GC by air at ambient relative humidity and
temperature.
Figure 4.3.2. Ambient temperature dimethylnitrosamine
storage test.
Figure 4.6. Typical chromatogram for
dimethylnitrosamine.
5. References
5.1. D.H. Fine, F.P. Roundbehler, E.D. Pellizzari, J.E. Bunch, R.W.
Berkley, J. McCrae, J.T. Bursey, E. Sawicki, K. Krost and G.A.
DeMarrais, N-Nitrosodimethylamine in Air, Bulletin of
Environmental Contamination and Toxicology, 15(6),
739-746 (1976).
5.2. D.H. Fine, D.P. Roundbehler, E. Sawicki, and K. Krost,
Determination of Dimethylnitrosamine in Air by Thermal Energy
Analysis: Validation of Analytical Procedures, Environmental
Science and Technology, 11(6), 577-80 (1977).
5.3. E.D. Pellizzari, J.E. Bunch, J.T. Bursey, and R.E. Berkley,
Estimation of N-Nitrosodimethylamine Levels in Ambient Air by
Capillary Gas-Liquid Chromatography/Mass Spectrometry, Analytical
Letters, 9(6), 579-594 (1976).
5.4. D.H. Fine, Analysis of N-Nitroso Compounds Using the Thermal
Energy Analyzer, Submitted to: International Agency for Research on
Cancer (IARC), Manual of Methods for Environmental Carcinogens, Volume
on N-Nitroso Compounds, Nov. 1976.
5.5. R.W. Fisher, R.W. Reiser, and B.A. Lasoski, Determination of
Sub-Microgram per Cubic Meter Levels of
N-Nitrosodimethylamine in Air, Analytical Chemistry,
49(12), 1821-1823 (1977).
5.6. D.H. Fine, D.P. Roundbehler, and N.P. Sen, A Comparison of
Some Chromatographic Detectors for the Analysis of Volatile
N-Nitrosamines, Agricultural and Food Chemistry,
24(5), 980-984 (1976).
5.7. P. Issenberg and H. Sornson, A Monitoring Method for Volatile
Nitrosamine Levels in Laboratory Atmospheres, Environmental N-Nitroso
Compounds Analysis and Formation, E.A. Walker and P. Bogovski, Eds.,
Lyon, France; IARC scientific Publication No. 14, (1976).
5.8. W. Fiddler, R.C. Doerr, J.R. Ertel, and A.E. Wasserman,
Gas-Liquid Chromatographic Determination of
N-Nitrosodimethylamine in Ham, Journal of the
AOAC, 54(5), 1160-1163 (1971).
5.9. D.H. Fine, P. Lieb, and F. Rufeh, Principle of Operation of
the Thermal Energy Analyzer for the Trace Analysis of Volatile and
Non-Volatile N-Nitroso Compounds, Journal of Chromatography,
107, 351-357 (1975).
5.10. "Registry of Toxic Effects of Chemical Substances", 1976
Edition (H.E. Christensen, E.J. Fairchild, Eds., B.S. Carroll, R.J.
Lewis, project Coordinators) U.S. Department of Health, Education, and
Welfare Public Health Service, Center for Disease Control, National
Institute for Occupational Safety and Health, U.S. Government Printing
Office, Washington, D.C. (1976).
5.11. P.N. Magee, and J.M. Barnes, Carcinogenic Nitroso Compounds,
Advances in Cancer Research, 10, 163-247 (1967).
5.12. P.N. Magee, Toxicity of Nitrosamines: Their Possible Human
Health Hazards, Fd. Cosmet. Toxicol., Great Britain, 9,
207-218 (1971).
5.13. J. March, Advanced Organic Chemistry: Reactions, Mechanisms,
and Structure, McGrawHill Book Company, New York, 486 (1968).
5.14. J.N. Pitts, P. Grosjean, K.V. Cauwenberght, J.P. Schmid, and
D.R. Fitz, Photooxidation of Aliphatic Amines under simulated
Atmospheric Conditions: Formation of Nitrosamines, Nitramines, Amides
and Photochemical Oxidant, Environmental Science and
Technology, 12(8), 946-953 (1978).
5.15. D. Shapley, Nitrosamines: Scientists on the Trail of Prime
Suspect in urban Cancer, Science, 191, 268 (1976).
5.16. Criteria Document: Recommendations for Occupational Exposure
standards for the Oxides on Nitrogen (Nitrogen Dioxide and Nitric
Oxide), U.S. Department of Health, Education, and Welfare, Public
Health Service, Center for Disease Control, National Institute for
Occupational Safety and Health, U.S. Government Printing Office,
Washington, D.C., 72-74 (1976).
5.17. B. Spiegelhalder, G. Eisenbrand, and R. Preussmann,
Contamination of Amines with N-Nitrosamines, Angew.
Chem. Int. Ed. Engl., 17(5), 367-368 (1978).
5.18. IARC Monographs on the Evaluation of Carcinogenic Risk of
Chemicals to Man, Vol. 1, International Agency for Research on Cancer,
Lyon, France, World Health Organization, 95106 (1971).
5.19. W. Lijinsky, S.S. Epstein, Nitrosamines As Environmental
Carcinogens, Nature, (London), 225, 21-23 (1970).
5.20. Carcinogens-Regulation and Control, U.S. Department of
Health, Education, and Welfare, Public Health Service, Center for
Disease Control, National Institute for Occupational safety and
Health, U.S. Government Printing Office, Washington, D.C., 46 (1977).
|